Assessment of aluminium level in commercial pasteurized and UHT milk in Bangladesh and their potential health risks
Abstract
Aluminium (Al) contamination in the dairy industry is an emerging concern due to potential health risks. With the increasing consumption of pasteurized and UHT milk in Bangladesh, monitoring Al levels has become crucial. This study aimed to measure Al concentrations in these processed products using inductively coupled plasma mass spectrometry (ICP-MS) and assess the associated health risks. Milk samples from 10 brands, including three different production lots, were collected from supermarkets. The instrumental precision for Al was 0.0212 mg/kg as a limit of detection (LOD) and 0.0643 mg/kg as a limit of quantification (LOQ), with a relative standard deviation (RSD) of 3.2%. The multi-element standard containing Al exhibited linearity in the concentration ranged from 0-0.5 mg/kg with a correlation coefficient value of 0.9994. Among the 30 samples analyzed, 13, including both pasteurized and UHT milks, were contaminated with Al. The mean Al concentrations in the contaminated samples were 0.46±0.5, 0.71±0.6, and 0.47±0.4 mg/kg in lots 1, 2, and 3, respectively, indicating variation across production lots. The estimated daily Al intake ranged from 2.00E-03 to 3.00E-03 mg/kg body weight, contributing 1.4 to 2.1% of the provisional tolerable weekly intake. The target hazard quotient (THQ) for the contaminated samples ranged from 0.045 to 0.070 (THQ < 1), suggesting that the Al levels pose no significant health risk. Thus, the Al content in pasteurized and UHT milk may be considered safe for consumption, albeit with the limitation of a small sample size in this study.
INTRODUCTION
Aluminium (Al) constitutes approximately 8.0% of the Earth's crust, making it the third most abundant element in the environment [1,2]. The element is naturally found in soil, minerals, rocks, water, and food [2]. However, despite its prevalence, Al does not fulfill any essential biological function in the human body rather linked to various health risks as reviewed by Klotz et al. [3]. The ubiquitous presence of Al in everyday products prompts ongoing investigations into its potential impact on human health [4]. In addition, the free metal cation of Al, which has high biological reactivity, is unnecessary and essentially toxic. People are consistently exposed to Al through different sources such as vaccinations, antacids, antiperspirants, and food, and this exposure is linked to inflammatory responses, oxidative damage, and disruptions in iron metabolism [5]. Chronic exposure to Al has raised concerns about its potential association with neurodegenerative diseases, including Parkinson's and Alzheimer's [6]. Beyond neurotoxicity, Al exposure has been linked to several other health issues such as bone injury, respiratory disorders, kidney damage, and potential carcinogenic effects [7]. Published research frequently indicates that diet is the primary source of Al exposure, with gastrointestinal absorption being the main pathway for Al entering the body [8]. The era of Al exposure has revealed that while chronic Al toxicity is not inevitable, it remains a potential risk. The extent of Al toxicity depends on their forms, exposure routes, and mode of delivery to the target sites [4]. This risk requires rigorous investigation and should not be underestimated.
Al is a widespread contaminant in the food industry, primarily due to its extensive use in food additives, packaging, and cooking equipment [9]. Furthermore, Al can naturally occur in certain foods, including vegetables, cereals, and dairy products [10]. Al-containing additives are commonly used to enhance the texture and appearance of many processed foods. These include anticaking agents and colorants, which can significantly increase Al levels in food products [9,10]. The use of Al foil and cookware can lead to the leaching of Al into food during cooking or storage. Foods that are acidic or salty can exacerbate this leaching process [11]. In Bangladesh, Al cookware is commonly used due to its affordability and good heat conduction properties. Studies indicate that approximately 97.56% of people use Al utensils, which can leach Al into food, especially during cooking processes involving acidic or salty foods [12].
Consumer preference for milk and milk products that provide superior nutrition, high biological value, and assurance of no associated health risks has been increasing [13]. The mineral content of milk is considered a direct indicator of the milk's hygienic quality and an indirect indicator of the environmental contamination at the production site [14]. Milk can be contaminated both before and after processing. Consequently, various toxic elements, such as Al, may be present in milk and milk products, leading to serious health issues for infants, children, and the elderly [15]. Several studies have reported that Al concentration in milk and milk products ranges from 0.003 to 3.4 mg/kg [16-20]. Al can enter milk through various sources, including the feed and fodder provided to animals, during the processing stage or through contamination from equipment made of metal [21]. The Al levels in milk can increase if aluminum-based utensils are used at any stage of milk processing or storage [22]. The leaching of Al from utensils depends on the quality of the containers, the pH levels maintained, the conditions during preparation, and the presence of chelating agents [23]. Pasteurized milk undergoes a heating process of 72°C for 15 seconds (High Temperature Short Time Pasteurization) or 63°C for 30 minutes (Vat Pasteurization). In contrast, ultra-high temperature (UHT) milk is subjected to 138°C for 2 seconds. Pasteurization can potentially increase Al leaching into milk, particularly if high temperatures, acidic conditions, or Al-containing equipment are involved. The human body can excrete small amounts of Al very efficiently. The Joint FAO/WHO Expert Committee on Food Additives (JECFA) has set a provisional tolerable weekly intake for Al at 2 mg/kg body weight [24]. In contrast, the European Food Safety Authority (EFSA) has established a lower tolerable weekly intake of 1 mg/kg body weight per week [25]. Therefore, the current study aimed to quantify the Al content in commercially available pasteurized and UHT milk in Bangladesh, following the validation of the analytical method. Furthermore, it intended to estimate the daily Al intake (DAI) and target hazard quotient (THQ).
MATERIALS AND METHODS
Sample collection
Commercially available pasteurized and UHT milk from 10 different brands were collected from supermarkets located in Dhaka and Gazipur district and labeled as A, B, C, D, E, F, G, H, I, and J. Of these, eight samples were pasteurized, and two were UHT milk. The milk samples of similar brands were further collected from analogous shops at three different times to confirm the differences in the production lot of the target samples. Thus, a total of 30 milk samples were obtained. After procuring, the milk samples were transferred to the laboratory in their original packaging using an ice box, appropriately labeled, and stored in a laboratory refrigerator (4°C). The samples were then analyzed within the recommended storage period.
Instrumentation
The quadrupole inductively coupled plasma-mass spectrometer (ICP-MS) used in this study was a NexION 1000 (PerkinElmer Inc., Waltham, USA). The system was equipped with a high-efficiency sample introduction desolvating system featuring a quartz cyclonic spray chamber. Additionally, it included a mixing chamber designed to further homogenize and stabilize the sample aerosol stream, ensuring a more consistent signal from the ICP-MS. The system employed a PFA-ST nebulizer operating with a peristaltic pump. Before each experiment, the instrument was tuned for optimal performance as recommended by the manufacturer. The instrument settings and operating conditions are detailed in Table 1.
Table 1. Parameters of ICP-MS for milk analysis.
Preparation of rinse solution, calibration standard, and blank
A rinse solution was prepared for the autosampler rinse port, consisting of 20 ml of ultrapure nitric acid (60%; Suprapur, Supelco, Sigma-Aldrich Chemie GmbH, Taufkirchen, Germany), 20 ml of Tergitol solution (70%; Sigma-Aldrich Chemie GmbH, Taufkirchen, Germany), and ultrapure water (Smart2Pure Water Purification System, Thermo Fisher Scientific, Budapest, Hungary) added to a final volume of 1000 ml. All calibration standard solutions were prepared from a 10 mg/l multi-element standard solution (Periodic table mix 1 for ICP, Sigma-Aldrich Chemie GmbH, Taufkirchen, Germany). In order to prepare 1 mg/l or 1000 µg/l stock solution, 1 ml of certified standard solution was mixed with 9 ml of 5% nitric acid. The standard solutions of varying concentrations (std 1, std 2, std 3, std 4, std 5, std 6) were subsequently prepared by diluting the stock solution with 5% nitric acid to a final volume of 10 ml, as shown in Table 2. For the standard blank solution, only 5% of nitric acid was used.
Table 2. Composition of standard solutions.
Sample preparation and digestion
During sample preparation, the milk samples were taken out from the refrigerator to equilibrate to room temperature. The Microwave Sample Preparation System (Titan MPS, PerkinElmer, Inc., Waltham, USA) with 75 ml vessels was used for the digestion process. Initially, 3 ml of each milk sample was placed into separate vessels in triplicate. Subsequently, 5 ml of ultrapure concentrated nitric acid (60%; Ultrpur, Sigma-Aldrich Chemie GmbH, Taufkirchen, Germany) and 2 ml of 30% hydrogen peroxide (Sigma-Aldrich Chemie GmbH, Taufkirchen, Germany) were added to each vessel. The sample blank solution was prepared by combining 5 ml of ultrapure concentrated nitric acid, 3 ml of ultrapure water, and 2 ml of 30% hydrogen peroxide. The vessels were then sealed and placed in a microwave digester, where a specific heating program (as outlined in Table 3) was applied for the digestion of the milk samples. The temperature was gradually increased from 140°C to 195°C, followed by a gradual decrease to 50°C. After placing the sample into the digester, the digestion process was completed within 90 min. Upon completion of digestion, the samples were allowed to cool for a designated period in a fume hood. The vessel caps were then gradually unscrewed to release any built-up pressure, after which the caps were fully removed.
Table 3. Temperature program for microwave digestion.
Analysis of samples
Prior to analysis, all digested samples were carefully filtered (Whatman filter paper, No. 1, Cytiva, Buckinghamshire, UK) to minimize contamination and transferred to 50 ml volumetric tubes. In accordance with the design, the instrument was turned into the appropriate “Standard” mode to achieve optimum sensitivity. All the standards, blanks, and samples were marked properly and then placed into the test tube holder. The digested milk samples were analyzed directly without undergoing any further dilution. A total of 30 samples and six standards, along with corresponding blanks, were analyzed using standard mode. The blank solution and standard solutions were also analyzed at regular intervals to observe the drift. The calibration standards were followed by the rinsing process, standard blank checks, sample blank checks, and, subsequently, the analysis of the milk samples.
Validation of method
Linearity, accuracy, and precision were used for method validation. Calibration curves were prepared using standard solutions at various concentrations (0, 1, 20, 100, 300, 500 µg/l) and analyzed with an ICP-MS instrument. A standard curve for Al was subsequently constructed, from which the R² value and the linear regression equation were derived. After running the 6 standards on ICP-MS, the standard calibration curves of Al were generated. As shown in Figure 1, Al had a good linear relationship with R2 value of 0.9994.
The precision of the instrument and sample digestion was assessed by determining the limits of detection (LOD), limits of quantification (LOQ), precision, and recovery percentages. The LOD and LOQ were calculated using the equations LOD = 3.3 × N/B and LOQ = 10 × N/B, where 'N' represents the standard deviation of intercept of Al (n = 3), and 'B' is the slope of the corresponding calibration curve. The instrumental precision analysis, as shown in Table 4, revealed a LOD for Al at 0.0212 µg/kg. The LOQ for Al was determined to be 0.0643 µg/kg (Table 4). Additionally, the relative standard deviation (RSD%) was calculated to be 3.2%. The method demonstrated efficiency in recovering the spiked Al, with a mean recovery of 100.8% (Table 4). These results were derived from the mean of six replicates, thereby ensuring the reliability and reproducibility of the method employed for determining Al in milk samples.
Table 5 illustrates the method validation results for the determination of Al in milk samples through the analysis of the precision of the digestion method.
The average concentration of Al detected in a milk sample with five replications was 0.38 ± 0.5 mg/kg. The addition of 0.2 mg/kg Al to the milk samples resulted in a mean spiked concentration of 0.58 ± 0.5 mg/kg. The average concentration of Al recovered from the spiked samples was 0.57 ± 0.5 mg/kg, with an average recovery percentage of 98.83 and an RSD value of 2.2%.
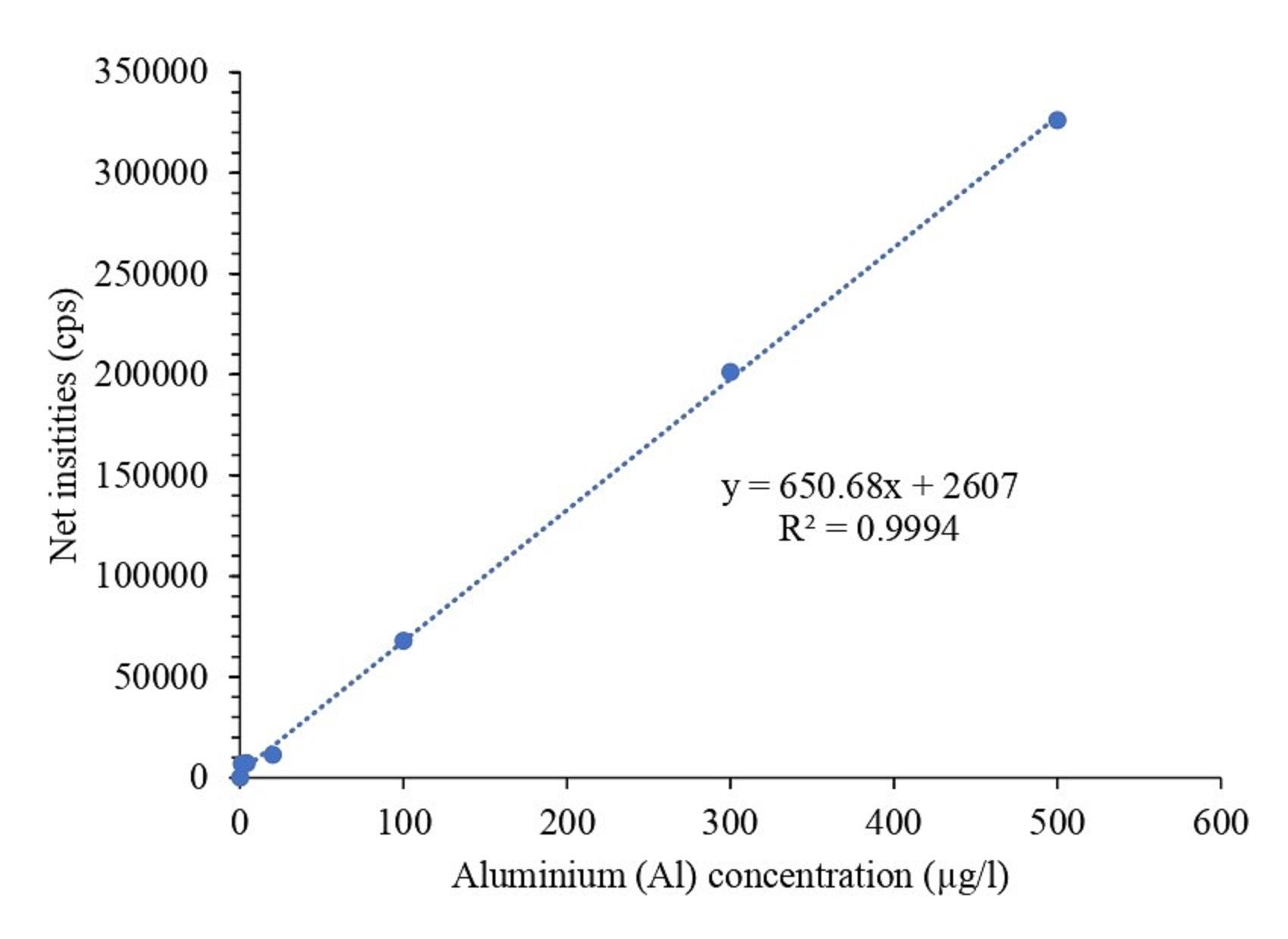
Table 4. Method validation through instrumental precision analysis.
Table 5. Method validation through analyzing the precision of the digestion method.
Assessment of human health risk
The human health risk assessment was performed as described in the published literature [26]. The daily Al intake (DAI), weekly Al intake (WAI), and THQ were calculated by the following equa:
Milk availability in Bangladesh was reported to be 234.45 ml per day per capita [27]. The average body weight of adults in Bangladesh is 55 kg [28]. The THQ was calculated using DAI and Referred oral dose (RfDo). A THQ value of less than 1 indicates that the exposed population is considered to be within a safe range. The RfDo for Al was not available; however, the Environmental Protection Agency (EPA) proposed a value of 0.043 mg/kg/day body weight for Al phosphide [2], which was used to calculate THQ. The RfDo for Al is not available; however, the EPA has proposed a value of 0.043 mg/kg/day for Al phosphide, which was used in the calculation of the THQ. Since the health risk results presented in the study were entirely based on calculated values, ethical approval was not sought during the study.
Statistical analysis
The data generated in this study were recorded in an open-source tabulation software. One-way analysis of variance (ANOVA) with Duncan's Multiple Range Tests (DMRT) among means was performed using an add-in software, yStat 2008 [Shinya Yamazaki, Koriyama, Japan], followed by comparisons. All statistical tests were two-tailed, and a p-value of less than 0.05 was considered statistically significant.
RESULTS
Presence of Al concentrations in milk samples
The average Al concentration in milk samples from 10 brands (A–J) across three separate production lots is depicted in Table 6. Among 30 samples, 17 had no detectable Al, while others showed varying levels of Al concentration (0.05-1.74 mg/kg, on a fresh basis). Al was not detected in samples A, B, E, F, and G across all production lots. However, sample H from production lot-2 exhibited an Al concentration of 0.07 mg/kg, with an RSD value of 7.6%. Considering the Al concentration across all three production lots, brand C exhibited the highest Al concentration (1.12-1.74 mg/kg), followed closely by brand D (0.51-0.95 mg/kg), with no statistically significant differences observed (p < 0.05). Brand J (0.09-0.46 mg/kg) displayed the second highest concentration, while brand H (0.00-0.07 mg/kg) recorded the lowest Al concentration. The Al concentration in brand I (0.07-0.32 mg/kg) did not significantly differ from that of brands H and J. A comparison of Al concentrations among milk samples from different brands within individual production lots revealed that samples from brand C had the highest Al concentration, followed by those from brand D. Although a significant difference was observed between the two brands, no significant difference was detected in their overall Al concentrations (Table 6). The Al concentrations in milk samples from brands I and J showed a significant difference in production lot-2 and lot-3. However, no significant variation in Al concentration was observed between the samples from these brands in lot-1. Al was detected in brand H exclusively in lot-2 and differed significantly from other brands (Table 6).
Table 6. Measured Al concentrations in milk samples from various brands and production lots.
Profile of Al contaminated milk samples and their health risk
The profile of Al-contaminated milk samples, along with their associated human health risks, was presented in Table 7. A total of 13 samples represented five brands and three production lots were identified as Al-contaminated. In Lot-1, 40% of the samples from brands C, D, I, and J were found to be contaminated with Al. The Al concentration ranged from 0.07 to 1.3 mg/kg, with a mean concentration of 0.46 ± 0.5 mg/kg. The DAI was calculated as 0.002 mg/kg body weight. The contribution to the Provincial Tolerable Weekly Intake (PTWI) was 1.37%, and the THQ value was determined 0.045. In contrast, Lot-2 exhibited contamination in five out of ten samples (50%), specifically from brands C, D, H, I, and J. The Al concentration in the contaminated samples ranged from 0.07 to 1.8 mg/kg, with a mean of 0.71 ± 0.6 mg/kg. The DAI from these contaminated samples was determined to be 0.003 mg/kg body weight. The contribution to the PTWI was 2.12% and the THQ value for Al in these samples was calculated to be 0.07. Similar to lot-1, 40% of the samples from brands C, D, I, and J in Lot-3 were found to be contaminated with Al. The calculated mean concentration of Al was 0.47 ± 0.4 mg/kg, with a range from 0.07 to 1.2 mg/kg. The DAI was calculated as 0.002 mg/kg body weight. The contribution to the PTWI was 1.4% and the THQ value was determined 0.047. In Lot-1, 40% of the samples from brands C, D, I, and J were identified as contaminated with Al. In Lot-2, contamination was observed in five out of ten samples (50%), specifically from brands C, D, H, I, and J. Similar to lot-1, 40% of the samples from brands C, D, I, and J were found to be contaminated with Al in lot-3.
Table 7. Profile of Al contaminated milk samples and their health risk.
DISCUSSION
A recent review covering articles published between 2000 and 2021 indicates that 63% of the studies use microwave-assisted digestion, and 40% employ ICP-MS to analyze milk and dairy products [29]. Validation of an analytical method is a critical step to ensure the accuracy and reliability of results. In this study, the method used to measure Al concentration in pasteurized and UHT milk samples is validated by assessing the precision of both the instrument and the digestion procedure. Additionally, spike and recovery tests, which are essential for evaluating the accuracy of analytical methods [30], are performed as part of the validation process. Our study demonstrates that the instrument can accurately detect a minimum Al concentration of 0.0212 mg/kg (LOD) and reliably quantify concentrations as low as 0.0643 mg/kg (LOQ), with an RSD of 3.2%. The high mean spiked recovery rate (100.8 and 98.8%) achieved for instrumental and digestion precision analysis in this study confirms the reliability and reproducibility of the method used for determining Al concentrations in milk samples. A comparison of the current findings with previously published studies indicates that the LOD and LOQ for Al in milk and dairy products range from 1.2 to 500 and 4.0 to 1000 µg/l or µg/kg, respectively [16-18], which are higher than those observed in our study. This suggests that the method employed in our study demonstrates a higher precision. The variations in LOD and LOQ values across different studies may be due to the instrumentation and conditions of the analysis. The average Al concentrations in 30 milk samples analyzed in the present study is 0.31 mg/kg. Several studies have reported Al concentrations in milk using ICP-MS. These studies reveal Al concentrations in cow's milk from China, Egypt, Italy, and Turkey, ranging from 0.00293 to 0.493 mg/kg [16-19].
Additionally, a recent systematic review [20] of studies published between 1993 and 2021 indicates that Al concentrations in pasteurized and sterilized milk from China, Egypt, Pakistan, Spain, and Turkey as determined by ICP-MS, range from 0.06 to 3.35 mg/kg. Our study demonstrates that the DAI for the tested milk samples ranges from 0.002 to 0.003 mg/kg body weight, contributing 1.37 to 2.12% of the PTWI. Based on the RfDo of 0.043 mg/kg/day, the calculated THQ value ranges from 0.047 to 0.070. Al contamination in milk and dairy products can originate from multiple sources. Studies demonstrate that Al may enter the milk supply through contaminated feeds and fodder consumed by lactating cows, as well as during the processing and storage stages through the use of metal-based equipment [21,22]. In Bangladesh, there are no available reports on the Al content in feeds, fodder, and milk before and after processing. However, due to the widespread use of Al-based cookware in everyday activities, there is significant potential for Al leaching from utensils at various stages of milk production and processing. This may account for the high percentage (43%) of Al-contaminated pasteurized and UHT milk samples observed.
According to the Agency for Toxic Substances and Disease Registry (ATSDR) [2], a minimal risk level (MRL) of 1 mg Al/kg/day is established for both chronic (≥365 days) and intermediate (15–364 days) durations of oral exposure to Al. The estimated DAI from our study indicates a lower level of Al intake through pasteurized and UHT milk. Although there is no permissible level of Al in pasteurized milk, the Food and Drug Administration (FDA) sets a limit of 0.2 mg/l for bottled water [2]. The Egyptian Organization for Standards & Quality (EOSQ) reports a permissible level of 50 µg/l for Al, as cited by Amer et al. [19]. In contrast, the Turkish Food Codex stipulates that the maximum allowable Al concentration in food products should not exceed 1500 µg/kg [31]. The THQ value serves as an important metric for assessing the health risks linked to the intake of food contaminated with metals. This offers a measurable assessment of the possible non-carcinogenic impacts resulting from prolonged exposure to toxic substances, especially via dietary consumption. The findings of the current study revealed that a THQ value for Al in the milk samples analyzed is below 1, suggesting that the Al levels are within safe limits. Consequently, the concentrations of Al in these milk samples are unlikely to pose significant health risks to consumers. However, it is important to acknowledge the limitations associated with the relatively small sample size, which may affect the applicability of the results to a broader population.
CONCLUSIONS
The present study represents the report on Al concentrations in commercially available pasteurized and UHT milk in Bangladesh. The findings indicate that 43% of the tested milk samples were Al contaminated. However, based on the estimated DAI and THQ values, these samples are deemed safe for consumption. Despite this, even low levels of Al in milk could pose potential health risks with long-term, high-volume consumption. Therefore, it is crucial to monitor the sources of Al in dairy food matrices and perform regular assessments using validated methods to ensure consumer safety.
ACKNOWLEDGMENTS
The authors would like to thank the Research Management Wing of Bangabandhu Sheikh Mujibur Rahman Agricultural University (BSMRAU RMW-UGC Funded Project: ID 30, 2020-2023) for financial support and Mr. Sujan Kumar Das, Assistant Instrument Engineer (Electronics), Dr. M. A. Wazed Mia, Central Laboratory, BSMRAU for his technical support.
AUTHOR CONTRIBUTIONS
MMR and SA were involved in the conception and design of the experiments. IJ and MUH contributed to performing the experiments. KMA and MMR analyzed data. KMA, IJ, and SA contributed to drafting the article. SAMH, ASMS, and MMR contributed to revising it critically for important intellectual content. All authors made the final approval of the version to be published.
CONFLICTS OF INTEREST
There is no conflict of interest among the authors.
References
- [1]Rabinovich D. The allure of aluminium. Nature Chemistry. 2013; 5: 76.
- [2]ATSDR (2008). Toxicological profile for aluminum. Available at: https://www.atsdr.cdc.gov/toxprofiles/tp22.pdf (Accessed: 02 October 2024).
- [3]Klotz K, Weistenhöfer W, et al. The health effects of aluminum exposure. Deutsches Ärzteblatt International. 2017;114: 653.
- [4]Exley C. The toxicity of aluminium in humans. Morphologie. 2016; 329: 51-55.
- [5]Darbre PD, Mannello F, et al. Aluminium and breast cancer: Sources of exposure, tissue measurements and mechanisms of toxicological actions on breast biology. Journal of Inorganic Biochemistry. 2013; 128: 257-261.
- [6]Kawahara M and Kato-Negishi M. Link between aluminum and the pathogenesis of Alzheimer′ s disease: the integration of the aluminum and amyloid cascade hypotheses. International journal of Alzheimer’s disease. 2011; 2011: 276393.
- [7]Bonfiglio R, Scimeca M, et al. The impact of aluminum exposure on human health. Archives of Toxicology. 2023; 97: 2997-2998.
- [8]Shardlow E, Linhart C, et al. The measurement and full statistical analysis including Bayesian methods of the aluminium content of infant vaccines. Journal of Trace Elements in Medicine and Biology. 2021; 66: 126762.
- [9]FEHD (2009). Aluminium in food. Risk Assessment Studies, Report No.-35. Available at: https://www.cfs.gov.hk/english/programme/programme_rafs/files/RA35_Aluminium_in_Food_e.pdf (Accessed: 28 September 2024).
- [10]EUFIC (2021). Aluminium in food (Q&A): Sources, safety and regulations. Available at: https://www.eufic.org/en/food-safety/article/aluminium-qa (Accessed: 28 September 2024).
- [11]Dordevic D, Buchtova H, et al. Aluminum contamination of food during culinary preparation: Case study with aluminum foil and consumers’ preferences. Food Science & Nutrition. 2019; 7: 3349-3360.
- [12]ESDO (2020). A Report on Aluminum Cookware: A Major Source of Lead and other Toxic Metal Contamination in Bangladesh. Available at: https://esdo.org/report-on-aluminum-cookware-a-major-source-of-lead-and-other-toxic-metal-contamination-in-bangladesh/ (Accessed: 01 October 2024).
- [13]Licata P, Trombetta D, et al. Levels of “toxic” and “essential” metals in samples of bovine milk from various dairy farms in Calabria, Italy. Environment international. 2004; 30: 1-6.
- [14]González-Montaña JR, Senís E, et al. Cadmium and lead in bovine milk in the mining area of the Caudal River (Spain). Environmental monitoring and assessment. 2012; 184: 4029-4034.
- [15]González-Weller D, Gutiérrez AJ, et al. Dietary intake of aluminum in a Spanish population (Canary Islands). Journal of agricultural and food chemistry. 2010; 58: 10452-10457.
- [16]İslamoğlu F, Torul O, et al, Determination of some metal contents of cow’s milk in Akçabaat district of Trabzon by ICP-MS. Turkish Journal of Analytical Chemistry. 2020; 2.
- [17]Monteverde V, Camilleri G, et al. Heavy metal levels in milk and serum of dairy cows from different farms located near an industrial area. Animals. 2022; 12: 2574.
- [18]Chen L, Li X, et al. Analysis of 17 elements in cow, goat, buffalo, yak, and camel milk by inductively coupled plasma mass spectrometry (ICP-MS). RSC advances. 2020; 10: 6736-6742.
- [19]Amer AA, El-Makarem HS, et al. Lead, cadmium, and aluminum in raw bovine milk: Residue level, estimated intake, and fate during artisanal dairy manufacture. Journal of Advanced Veterinary and Animal Research. 2021;8: 454.
- [20]Alinezhad Z, Hashemi M, et al. Concentration of heavy metals in pasteurized and sterilized milk and health risk assessment across the globe: A systematic review. Plos one. 2024; 19: e0296649
- [21]Deeb AM and Gomaa GM. Detection of aluminium in some dairy products at Kafr-El-Sheikh, Egypt. Global Veterinaria. 2011; 6: 01-05.
- [22]Semwal AD, Padmashree A, et al. Leaching of aluminium from utensils during cooking of food. Journal of the Science of Food and Agriculture. 2006; 86: 2425-2430.
- [23]Al Juhaiman LA. Estimating Aluminum leaching from Aluminum cook wares in different meat extracts and milk. Journal of Saudi Chemical Society. 2010; 14: 131-137.
- [24]FAO/WHO (2012). Safety evaluation of certain food additives and contaminants. Available at: https://iris.who.int/bitstream/handle/10665/44521/9789241660648_eng.pdf?sequence=1 (Accessed: 24 September 2024).
- [25]European Food Safety Authority (EFSA). Safety of aluminium from dietary intake‐scientific opinion of the panel on food additives, flavourings, processing aids and food contact materials (AFC). EFSA Journal. 2008; 6: 754.
- [26]Kabir A, Khan K, et al. A study of heavy metal presence in cow milk of different dairy farms near Karnafuli paper mills, Chittagong, Bangladesh. American Journal of Engineering Research. 2017; 6: 329-333.
- [27]DLS (2024). Livestock Economy at a Glance. Available at: https://file-rajshahi.portal.gov.bd/uploads/0f236d9d-0a28-4fbd-b4d5-593734ed5892/670/9be/f6e/6709bef6e805f380007609.pdf (Accessed: 24 September 2024).
- [28]WHO (2010). Non-communicable disease risk factor survey Dhaka. Non-communicable disease risk factor survey Bangladesh 2010. Available at: https://cdn.who.int/media/docs/default-source/searo/bangladesh/pdf-reports/year-2007-2012/non-communicable-disease-risk-factor-survey-bangladesh-2010.pdf?sfvrsn=37e45e81_2 (Accessed: 27 September 2024).
- [29]Soares S, Moraes LM, et al. Sample preparation and spectrometric methods for elemental analysis of milk and dairy products–A review. Journal of Food Composition and Analysis. 2023; 115: 104942.
- [30]Thakur D, Dubey NP, Neeti Prakash Dubey, and Rajvinder Singh. A review on spike and recovery method in analytical method development and validation. Critical Reviews in Analytical Chemistry. 2022; 1-19.
- [31]Amer AA. Aluminum in artisanal dairy products: occurrence, residues level, estimated intake and target hazard quotient. 2019; 55-60.