Effect of lipoic acid on the expression of nonalcoholic fatty liver disease-associated genes in the liver of rats fed a hypercaloric choline-deficient diet
Abstract
Nonalcoholic fatty liver disease (NAFLD) is one of the most common chronic liver pathologies. Molecular mechanisms of NAFLD pathogenesis are complex and still require further clarification. Thus, this study aimed to investigate the impact of lipoic acid (LA), widely used as an antioxidant, on the development of different links of NAFLD, including de novo lipogenesis, antioxidant and xenobiotic-metabolizing enzymes by gene expression analysis. The experiment was carried out on three groups of male Wistar rats received control diet, hypercaloric choline-deficient diet (HCCD) or HCCD with LA (HCCD+LA). In the liver of rats the expression of acetyl-CoA carboxylase 1 (Acaca), fatty acid synthase (Fasn), stearoyl-CoA desaturase (Scd) and transcriptional regulators sterol-regulatory element-binding protein SREBP (Srebf1), carbohydrate response element-binding protein ChREBP (Mlxipl), peroxisome proliferator-activated receptor alpha PPARα (Ppara), heme oxygenase-1 HO-1 (Hmox1) and nuclear erythroid 2-related factor 2 Nrf2 (Nrf2), cytochrome P450 1A1 CYP1A1 (CYP1A1) and aryl hydrocarbon receptor AhR (AhR), cytochrome P450 2E1 CYP2E1 (CYP2E1) genes were evaluated. Supplementation of HCCD with LA led to an even greater than in HCCD group decrease in Scd gene expression by 88% (p<0.05), as well as a marked suppression of Ppara and Mlxipl by 37% (p<0.05) and 27% (p<0.05), to an 80% (p<0.05) elevation of Hmox1 gene expression relative to the HCCD group, had no pronounced effect on CYP1A1 and AhR gene expression but resulted in a 38% (p<0.05) suppression of CYP2E1 expression compared with that slightly elevated by HCCD. In conclusion, the current study showed that LA reduces lipogenesis de novo, restores the expression of Hmox1 gene of the antioxidant enzyme HO-1, diminished by the HCCD diet, and decreases HCCD-induced level of the expression of CYP2E1 gene of CYP2E1 enzyme, a potential source of free radicals.
INTRODUCTION
Nonalcoholic fatty liver disease (NAFLD) is one of the most common chronic liver pathologies. Pathogenesis of NAFLD is represented by complex mechanisms including insulin resistance which promote fat accumulation in hepatocytes and increase the vulnerability of hepatocytes for many factors such as oxidative stress, inflammation, activation of Kupffer cells, fibrogenesis and other. These processes lead to endoplasmic stress, oxidative stress, hepatocyte apoptosis and fibrosis [1,2]. Molecular mechanisms of NAFLD pathogenesis are complex and still require further clarification.
High fructose intake affects the progression and severity of NAFLD by promoting de novo lipogenesis and increasing insulin resistance, oxidative stress, inflammation and fibrosis [3]. The regulation of lipogenesis occurs mainly at the level of transcription. Glucose metabolites produced during glycolysis activate the transcription factors SREBP and ChREBP [4], which bind to carbohydrate response elements in the promoters of target genes Acaca, Fasn and Scd [5], which catalyze, respectively, the conversion of acetyl-CoA to malonyl-CoA and further to acyl-CoA followed by the formation of monounsaturated fatty acids. PPARα, which stimulates β-oxidation reactions of fatty acids, also plays an important role in lipid metabolism [6].
Fat accumulation causes metabolic abnormalities, leading to excessive mitochondrial reactive oxygen species (ROS) production and endoplasmic reticulum stress, which in turn can cause inflammation, cell damage and death. The main sources of ROS in the cells are mitochondria, peroxisomes, and cytochrome P450 [7, 8].
The antioxidant defense of the cell, regulated by the Nrf2/ARE pathway, counteracts the damage caused by oxidative stress by promoting the restoration of normal lipid metabolism [9]. High levels of ROS lead to the release of Nrf2 and its translocation into nucleus, which promotes the activation of transcription of antioxidant enzyme genes containing antioxidant responsive elements (ARE) in promoter region, one of such enzymes is HO-1 [10]. Oxidative stress markers are used to assess the pathological state and disease progression [11, 12].
Literature data indicate that lipoic acid (LA), a natural sulfur-containing compound, may have pronounced antioxidant activity [13]. This study aimed to investigate the impact of LA on the development of different links of NAFLD, including de novo lipogenesis, antioxidant and xenobiotic-metabolizing enzymes by gene expression analysis.
MATERIALS AND METHODS
Experimental protocol
All experimental procedures were performed according to the recommendations of the Council for International Organization of Medical Sciences and the International Council for Laboratory Animal Science, and adhered the standard principles described in “Guide for the Care and Use of Laboratory Animals” (8th edition). Ethical approval was obtained for this research from the Federal Research Centre of Nutrition and Biotechnology Ethics Committee (No. 7/24.06.2019).
Experimental design was described previously [14]. Study was carried out on three groups of male Wistar rats (n=8 of animals in each group). HCCD diet was used to simulate NAFLD. There was a control group fed a normal diet, HCCD group fed a HCCD diet and HCCD+LA group fed a HCCD diet supplemented with LA (Chem Impex International, Inc., Wood Dale, USA, Cat# 29862) in an estimated dose of 75 mg/kg of body weight. The average daily LA intake by animals calculated on the basis of the feed eaten throughout the experiment was 61 mg/kg body weight. The total duration of dietary feeding was 8 weeks. After 8 weeks of the experimental period, the animals were subjected to 16–18 h of fasting. After decapitation liver samples were collected and stored at -80˚C before RNA isolation.
Gene expression analysis
In the liver of rats, the expression of the genes, Acaca, Fasn, Scd, SREBP-1c (Srebf1), ChREBP (Mlxipl), PPARα (Ppara), HO-1 (Hmox1), Nrf2 (Nrf2), CYP1A1 (CYP1A1), AhR, CYP2E1 (CYP2E1), β-actin (Actb) and GAPDH (glyceraldehydes-3 phosphate dehydrogenase) (Gapdh) were evaluated by real-time reverse transcription polymerase chain reaction (RT-PCR). The samples of total RNA from liver tissue were isolated using TRI Reagent (Sigma-Aldrich, St. Louis, USA), and synthesis of complementary DNA (cDNA) was performed using MMLV RT kit (Evrogen, Moscow, Russia) according to the manufacturer’s protocol. The primers and probes for RT-PCR were provided by DNA Synthesis (Moscow, Russia) (Table 1). The 25 μl PCR reaction mixture contained 2.5 μl of cDNA (dilution 1:10), prepared in the reverse transcription reaction from 2 μg of total RNA, 2.5 μl of 10×Taq Turbo buffer for HS Taq DNA polymerase (with 2.5 mM MgCl2) (Evrogen, Moscow, Russia), 1 μl (F+R) primers (10 μM), 0.5 µl of FAM probe (10 µM), 1.0 µl of dNTPs mixture (10 mM) (Evrogen, Moscow, Russia), 0.25 µl of HS Taq DNA polymerase (5 units/µl) (Evrogen, Moscow, Russia), 17.25 µl of nuclease-free water (Thermo Scientific, USA). Amplification was performed on a CFX96 device (Bio-Rad, USA, made in Singapore). Gene expression was assessed by threshold cycle value and normalized relative to reference genes Actb and Gapdh by 2-ΔΔCt method [15].
Table 1. Primer and probe sequences in rats.
Statistical analysis
To establish statistically significance of the differences (p<0.05) between animal groups we used Kruskal-Wallis test and Dann multiple comparison criterion as a post hoc test. The calculations were performed using the statistical package of SPSS® 23.0 and Microsoft Excel for Windows.
RESULTS
Effect of LA and HCCD on the gene expression of key de novo lipogenesis enzymes
As shown in Figure 1, the expression of the genes of the key de novo lipogenesis enzymes Fasn and Acaca did not differ significantly in the rats received HCCD compared to the control group, but a significant suppression by 79% (p<0.05) of the expression level of Scd gene responsible for monounsaturated fatty acid synthesis was observed.
There was also no statistically significant effect of the experimental diet on the mRNA level of the Ppara gene (Figure 2A), the Srebf1 gene (Figure 2C) encoding the SREBP protein responsible for gene expression of de novo lipogenesis enzymes, the Mlxipl gene (Figure 2B) encoding the transcription factor ChREBP, which controls the gene expression of carbohydrate metabolism enzymes. However, supplementation of HCCD with LA led to an even greater decrease in Scd gene expression by 88% (p<0.05), as well as a marked suppression of Ppara and Mlxipl mRNA levels by 37% (p<0.05) and 27% (p<0.05), respectively, relative to the HCCD group.
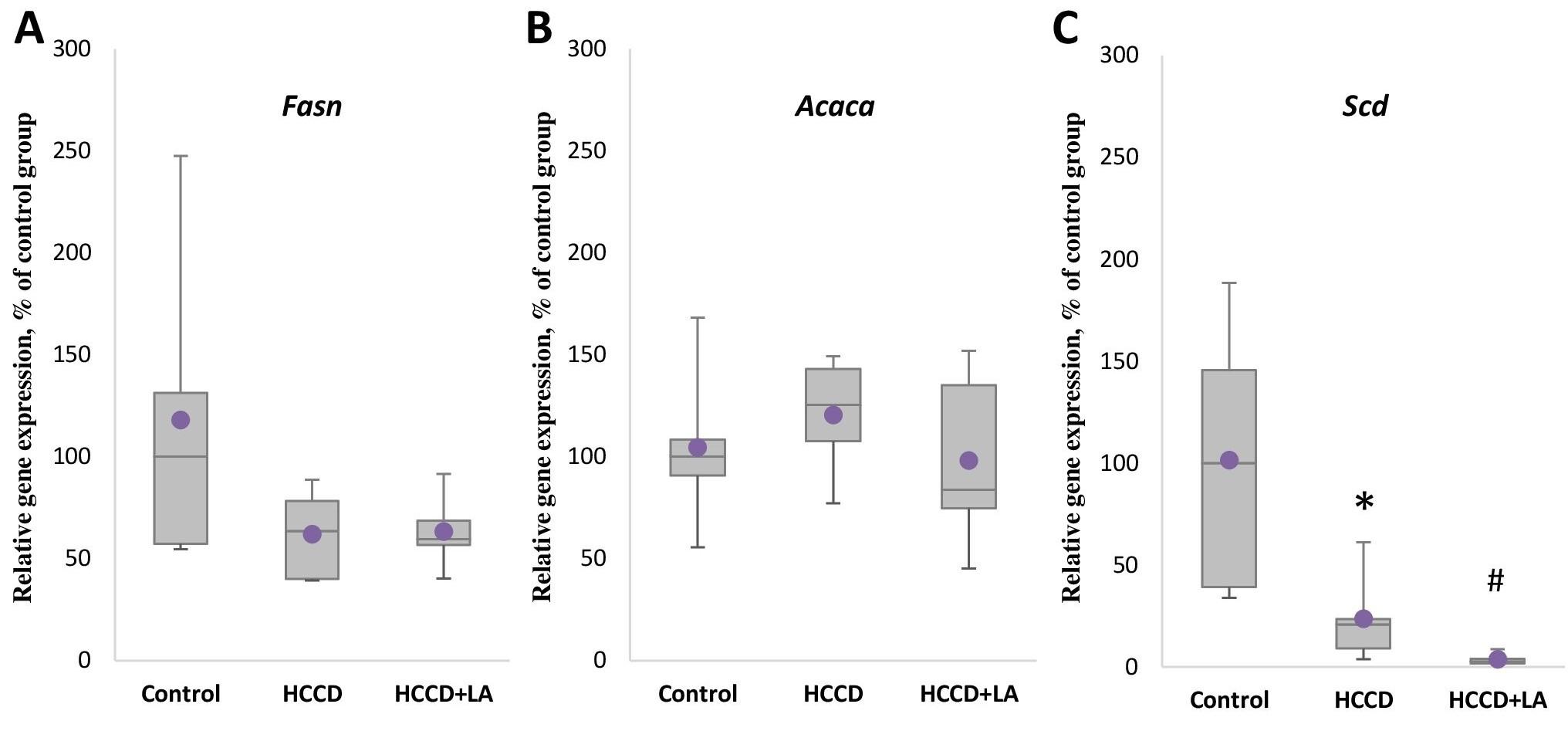
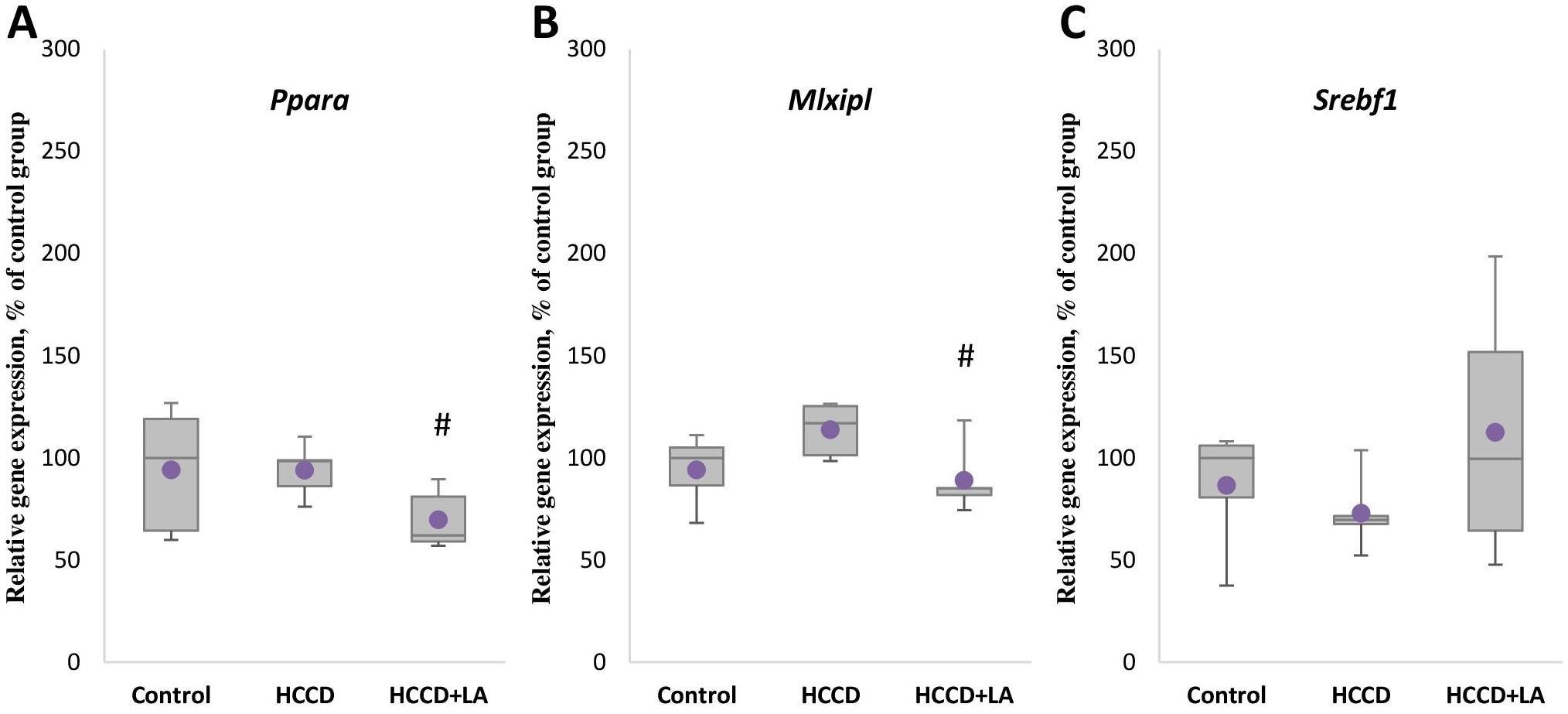
Effect of LA and HCCD on the gene expression of antioxidant enzyme HO-1
Although not statistically significant a decrease by 40% in the mRNA level of Hmox1 gene of the key antioxidant enzyme HO-1 (Figure 3A) was observed in animals receiving HCCD compared to the control group. The expression level of the transcription factor Nrf2 gene (Figure 3B) in this group (HCCD) increased by 18% relative to the control group, but also not significantly. In turn, enrichment of the experimental diet with LA led to an 80% (p<0.05) elevation of Hmox1 gene expression, relative to the HCCD group, and had no significant effect on Nrf2 mRNA levels.
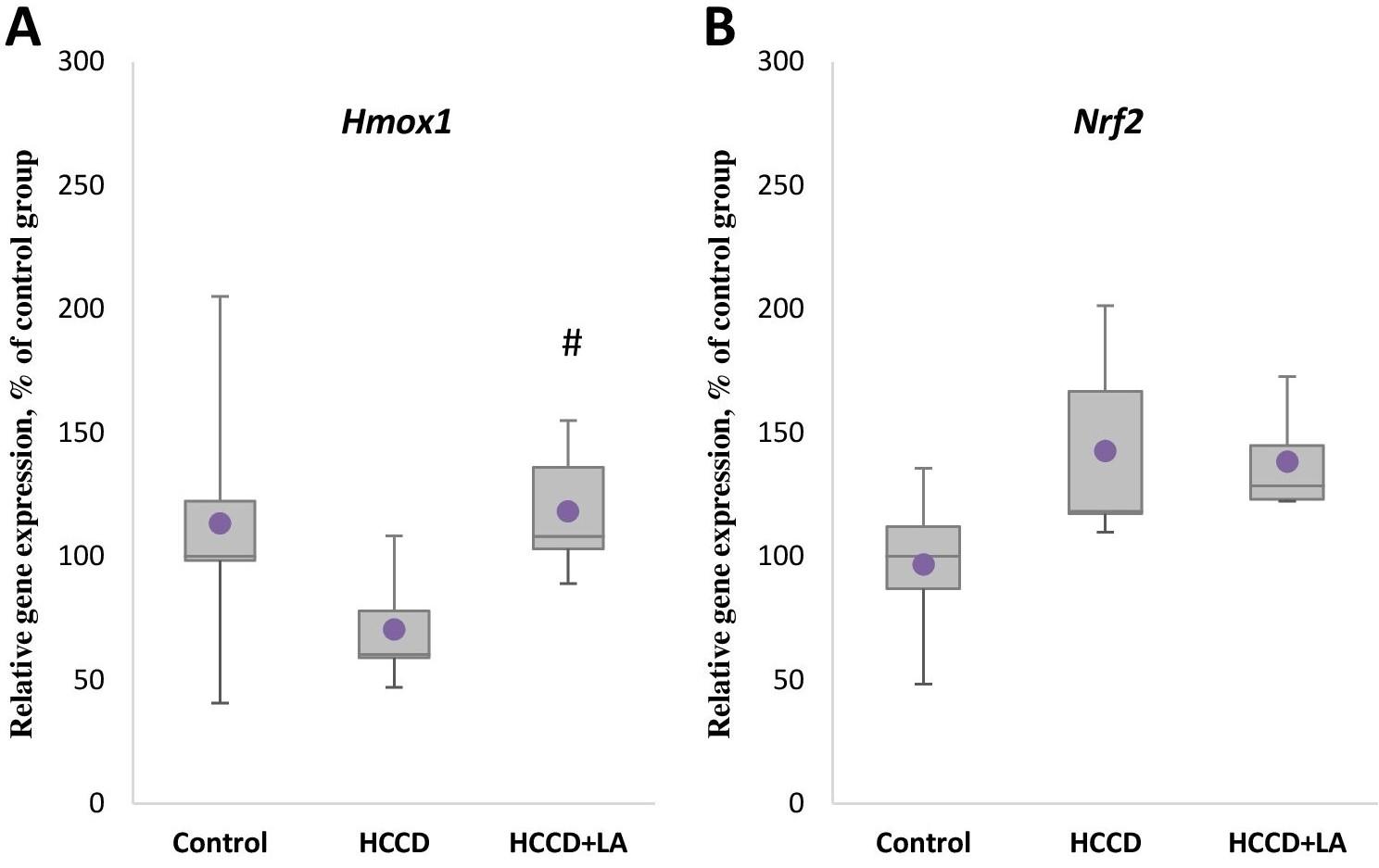
Effect of LA and HCCD on the gene expression of xenobiotic metabolizing enzymes
Analysis of the expression of the gene encoding the key xenobiotic metabolizing enzyme CYP1A1 showed that the level of CYP1A1 mRNA (Figure 4A) significantly decreased in the HCCD group by 83% (p<0.05) relative to the control group, while no effect of experimental diet on the expression of the transcription factor AhR (Figure 4B) regulated the expression of this enzyme was observed. The mRNA level of the CYP2E1 gene (Figure 4C) encoded the CYP2E1 enzyme, which is a potential source of free radicals, increased by 20% (p>0.05) in the group of animals receiving HCCD, although statistically insignificant. Supplementation of HCCD with LA had no pronounced effect on CYP1A1 enzyme gene expression and AhR transcription factor expression compared to the HCCD group. Consumption of LA as part of HCCD diet resulted in a 38% (p<0.05) suppression of CYP2E1 expression compared with that slightly elevated by HCCD.
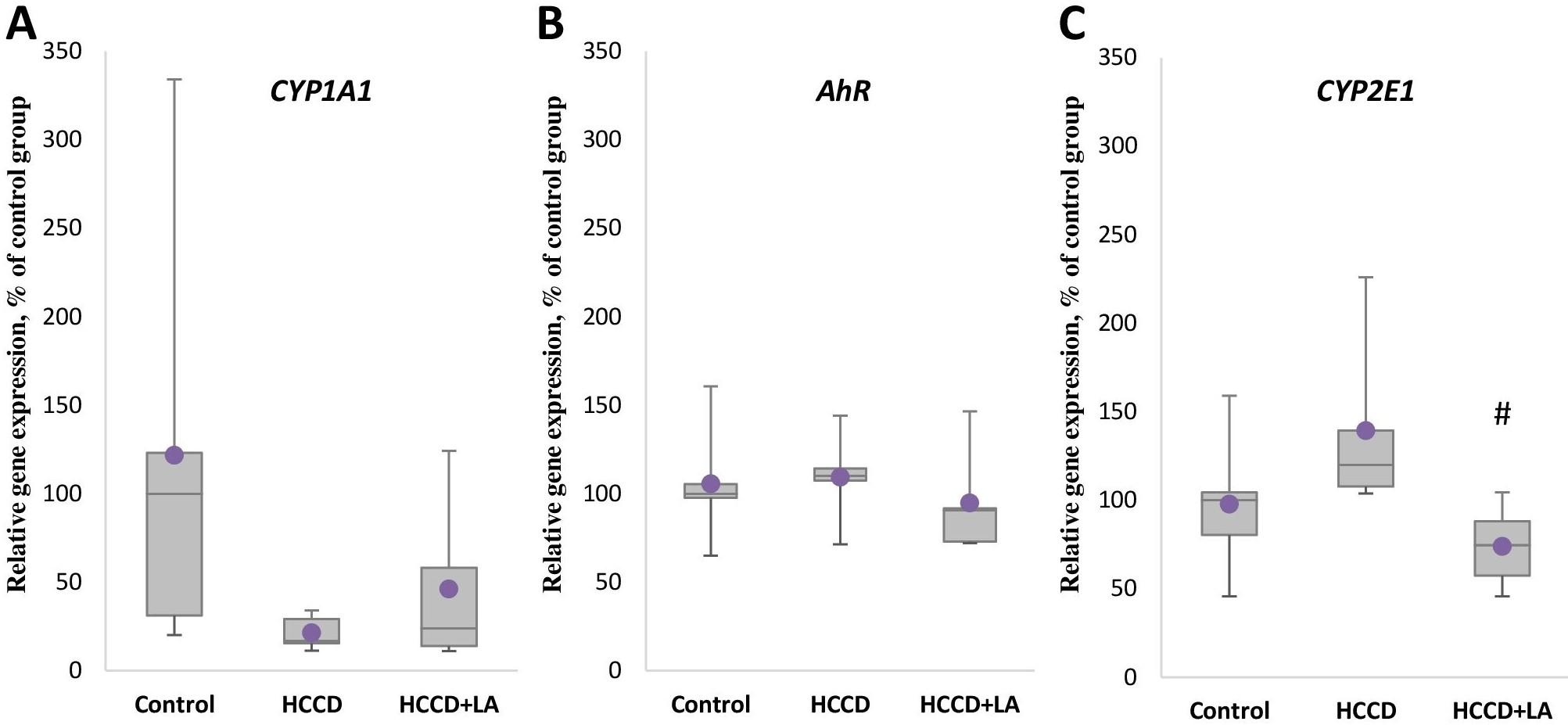
DISCUSSION
The previously published results obtained in this work showed that feeding of rats with HCCD for 8 weeks led to an increase in final body weight and in liver weight, which was associated with the accumulation of lipids in the liver and the development of steatosis in Wistar rats, which is one of the manifestations of NAFLD [14].
Gene expression analysis conducted here in our study revealed that supplementation of HCCD with LA led to decreasing in Scd gene expression, as well as a marked suppression of Ppara and Mlxipl mRNA levels relative to the HCCD group. Similar results to ours have been demonstrated in a number of papers. Thus, a study using human abdominal subcutaneous fat adipocytes showed a decrease in the protein levels of FASN, SCD (SCD is a key enzyme involved in the control of lipogenesis de novo, catalyzing the limiting step of monounsaturated fatty acid synthesis) and ACC (ACACA), a key enzyme of the lipogenic pathway that mediates the initial stage of fatty acid synthesis [16]. According to Guo et al. addition of α-lipoic acid to the diet (0.2%) for 5 weeks reduced Scd expression in the liver but increased Srebp1 expression at the mRNA level in Sprague-Dawley rats. In addition, supplementation with α-lipoic acid had no effect on hepatic Fasn mRNA levels but decreased hepatic FASN expression at the protein level in rats. The expression of carnitine-O-palmitoyltransferase 1a (Cpt1α) gene involved in fatty acid transport was unchanged [17]. Feeding of Zucker diabetic fatty (ZDF) rats with LA led to the suppression of the expression of the lipogenic genes Acaca, Fasn in liver and adipose tissue, reduced hepatic levels of ACC1/2, FASN proteins, and stimulated the expression of PPARa target genes, carnitine-O-palmitoyltransferase 1b (Cpt1b), but not Cpt1a [18]. In another study conducted on ZDF rats, feeding of ZDF rats with LA reduced the mRNA levels of ACC1 and FAS by 66% and 74% respectively, compared to animals fed the paired diet. Nuclear ChREBP protein was decreased in LA-treated livers, whereas nuclear SREBP-1c protein was not significantly altered [19]. Enrichment of the experimental diet with LA led to an elevation of Hmox1 gene expression, relative to the HCCD group, and had no significant effect on Nrf2 mRNA levels. Same result was observed in the study on the male Otsuka Long-Evans Tokushima Fatty (OLETF) rats, LA increased HO-1 expression in the liver [20]. Consumption of LA as part of HCCD diet resulted in a suppression of CYP2E1 expression compared with that slightly elevated by HCCD. Changes in the activity of CYP2E1 enzyme were observed in our previously published paper, the activity of microsomal CYP2E1 in the liver of rats in the HCCD group exceeded that in the control group by 22%, noteworthy there was a 45% inhibition of CYP2E1 activity in the liver of rats treated with LA compared to HCCD group [14]. Similar results were obtained in a study on C57BL6 mice using a methionine choline deficient diet (MCD). Thus, CYP2E1 expression in the liver of MCD mice was significantly higher than that of control mice, and LA inhibited the MCD diet-induced expression of CYP2E1 at the mRNA and protein levels [21].
CONCLUSIONS
Thus, our study showed that LA reduces lipogenesis de novo, restores the expression of Hmox1 gene of the antioxidant enzyme HO-1, diminished by the experimental diet (HCCD), and decreases HCCD-induced level of the expression of CYP2E1 gene of CYP2E1 enzyme, a potential source of free radicals (Figure 5). More detailed investigations are needed for further clarification of impact of LA and another biologically active compounds on the development of different links of NAFLD including studies on skeletal muscle and white adipose tissue.
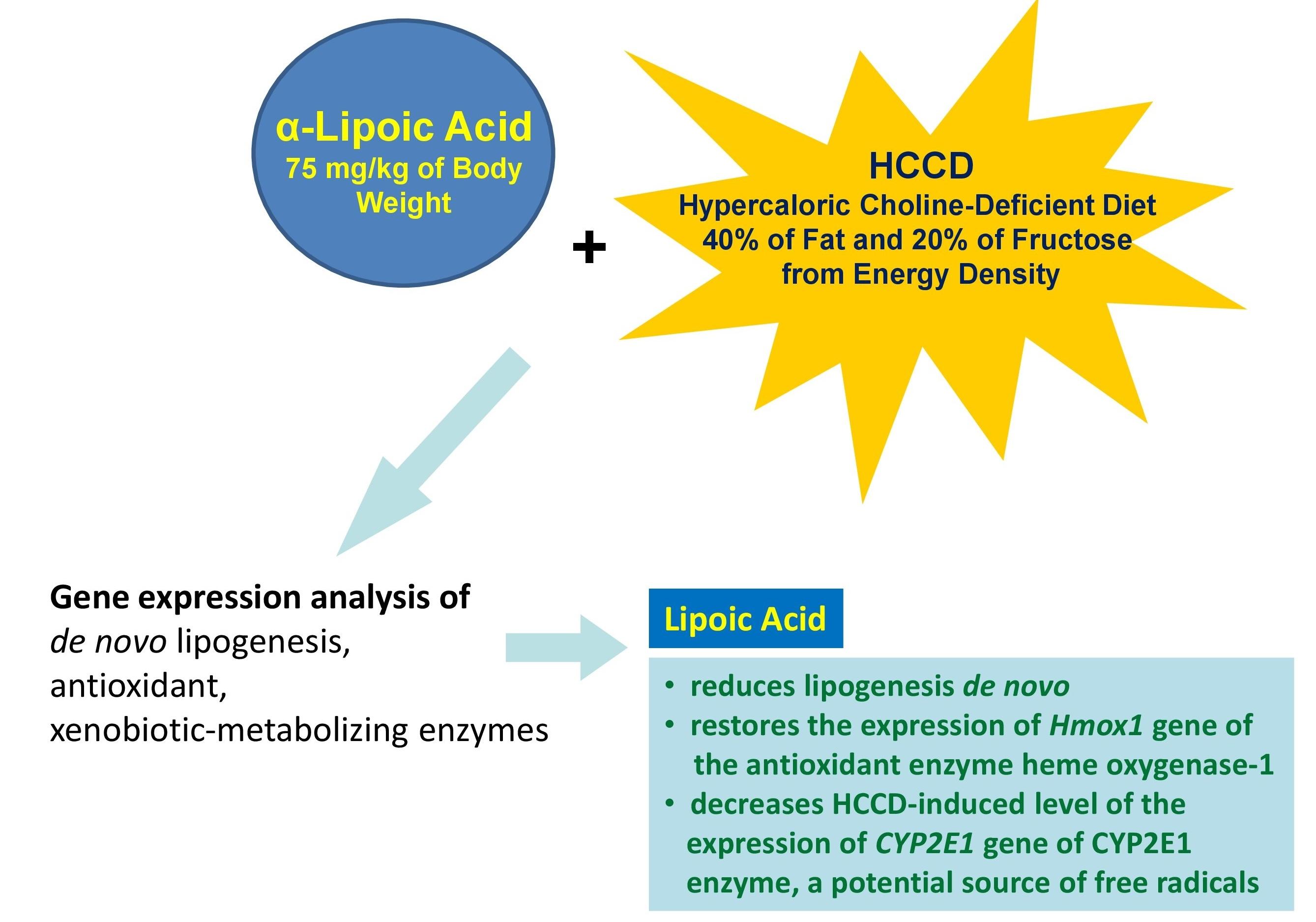
ACKNOWLEDGMENTS
This work was carried out at the expense of a subsidy for the implementation of a state task within the framework of the Basic Scientific Research Program (topic of the Ministry of Education and Science of the Russian Federation No. 0529-2019-0058).
AUTHOR CONTRIBUTIONS
AB: Data acquisition; data analysis/interpretation; statistical analysis; drafting manuscript. NT: Data acquisition; data analysis/interpretation; statistical analysis; critical revision of manuscript. VT: Concept and design; supervision; final approval. All authors read and approved of the final manuscript.
CONFLICTS OF INTEREST
There is no conflict of interest among the authors.
References
- [1]Masarone M, Rosato V, et al. Role of Oxidative Stress in Pathophysiology of Nonalcoholic Fatty Liver Disease. Oxid Med Cell Longev. 2018; 2018: 9547613.
- [2]Buzzetti E, Pinzani M, Tsochatzis EA. The multiple-hit pathogenesis of non-alcoholic fatty liver disease (NAFLD). Metabol. 2016; 65(8): 1038-1048.
- [3]Vos MB, Lavine JE. Dietary fructose in nonalcoholic fatty liver disease. Hepatology. 2013; 57(6): 2525-2531.
- [4]Linden AG, Li S, et al. Interplay between ChREBP and SREBP-1c coordinates postprandial glycolysis and lipogenesis in livers of mice. J Lipid Res. 2018; 59(3): 475-487.
- [5]Ortega-Prieto P, Postic C. Carbohydrate Sensing Through the Transcription Factor ChREBP. Front Genet. 2019; 10: 472.
- [6]Qiu YY, Zhang J, et al. Roles of the peroxisome proliferator-activated receptors (PPARs) in the pathogenesis of nonalcoholic fatty liver disease (NAFLD). Pharmacol Res. 2023; 192: 106786.
- [7]Jarukamjorn K, Jearapong N, et al. A High-Fat, High-Fructose Diet Induces Antioxidant Imbalance and Increases the Risk and Progression of Nonalcoholic Fatty Liver Disease in Mice. Scientifica (Cairo). 2016; 2016: 5029414.
- [8]Hwang I, Uddin MJ, et al. The impaired redox balance in peroxisomes of catalase knockout mice accelerates nonalcoholic fatty liver disease through endoplasmic reticulum stress. Free Radic Biol Med. 2020; 148: 22-32.
- [9]Arroyave-Ospina JC, Wu Z, et al. Role of Oxidative Stress in the Pathogenesis of Non-Alcoholic Fatty Liver Disease: Implications for Prevention and Therapy. Antioxidants (Basel). 2021; 10(2): 174.
- [10]Ngo V, Duennwald ML. Nrf2 and Oxidative Stress: A General Overview of Mechanisms and Implications in Human Disease. Antioxidants (Basel). 2022; 11(12): 2345.
- [11]Marrocco I, Altieri F, Peluso I. Measurement and Clinical Significance of Biomarkers of Oxidative Stress in Humans. Oxid Med Cell Longev. 2017; 2017: 6501046.
- [12]Vona R, Pallotta L, et al. The Impact of Oxidative Stress in Human Pathology: Focus on Gastrointestinal Disorders. Antioxidants. 2021; 10(2): 201.
- [13]Tibullo D, Volti GL , et al. Biochemical and clinical relevance of alpha lipoic acid: antioxidant and anti-inflammatory activity, molecular pathways and therapeutic potential. Inflamm Res. 2017; 66(11): 947-959.
- [14]Kravchenko LV, Aksenov IV, et al. Nutrients. Lipoic Acid Exacerbates Oxidative Stress and Lipid Accumulation in the Liver of Wistar Rats Fed a Hypercaloric Choline-Deficient Diet. 2021; 13(6): 1999.
- [15]Livak KJ, Schmittgen TD. Analysis of relative gene expression data using real-time quantitative PCR and the 2(-Delta Delta C(T)) Method. Methods. 2001; 25(4): 402-408.
- [16]Fernández-Galilea M, Pérez-Matute P, et al. α-lipoic acid reduces fatty acid esterification and lipogenesis in adipocytes from overweight/obese subjects. Obesity. 2014; 22(10): 2210-2215.
- [17]Guo Sh, Yan K , et al. α-Lipoic Acid Alleviates Hepatic Lipid Deposition by Inhibiting FASN Expression via miR-3548 in Rats. Nutrients. 2021; 13(7): 2331.
- [18]Yi X, Pashaj A, et al. Reversal of obesity-induced hypertriglyceridemia by (R)-α-lipoic acid in ZDF (fa/fa) rats. Biochem Biophys Res Commun. 2013; 439(3): 390-395.
- [19]Butler JA, Hagen TM, Moreau R. Lipoic acid improves hypertriglyceridemia by stimulating triacylglycerol clearance and downregulating liver triacylglycerol secretion. Arch Biochem Biophys. 2009; 485(1): 63-71.
- [20]Jung TS, Kim SK, et al. α-lipoic acid prevents non-alcoholic fatty liver disease in OLETF rats. Liver Int. 2012; 32(10): 1565-1573.
- [21]Min AK, Kim MK, et al. Alpha-lipoic acid attenuates methionine choline deficient diet-induced steatohepatitis in C57BL/6 mice. Life Sci. 2012; 90(5-6): 200-205.