Effect of geographical and agroclimatic location on phytocompounds and antioxidant activity of Moringa oleifera leaves
Abstract
Moringa oleifera L. is renowned for its rich phytochemical content and significant biological activity. The influence of geographic location and agroclimatic conditions on phytochemical content and bioactivity of plants remains unknown. Therefore, this study aimed to analyze the impact of geographical and agroclimatic location on the phytochemical content, antioxidant activity, and antibacterial potential of M. oleifera leaves. Phytochemical content was measured qualitatively and quantitatively, as well as bioactivity analysis was carried out by testing antioxidant and antibacterial activity. Interestingly, three dominant compounds were identified in both samples using the molecular docking tool. Phytochemical analysis revealed the presence of a broad spectrum of secondary metabolites in both samples, which were collected from two different locations. The identified metabolites were alkaloids, steroids, terpenoids, saponins, tannins, phenols, coumarins, flavonoids, quinones, and glycosides. The accumulation of phenols, flavonoid, and antioxidants in sample M1 was higher compared to sample M2. The results further suggest the confirmation of OH, C=O, CO, aliphatic, and aromatic carbons functional groups in samples. The most dominant compound 9,12,15-Octadecatrienoic acid (Z, Z, Z) found in sample M2. Molecular docking analysis suggests that the detected compounds have promising antioxidant and antibacterial activity, but their potential may vary due to divergence of geographical location and agroclimatic conditions. In addition, geographical location and agroclimatic conditions influenced the potential of other phytochemical and bioactivity in M. oleifera leaves. In conclusion, the overall findings can be effective for screening environmental factors-based regulation of potential compounds in M. oleifera and their pharmacological interest.
INTRODUCTION
Moringa oleifera L. locally known as Kelor in Indonesia, belongs to the Moringaceae family, and it is also known as the drumstick or radish tree globally. The plant grows in tropical and subtropical areas and at temperatures around 25–35°C. M. oleifera grows well in dappled sunlight but cannot tolerate waterlogged soils. Plant optimal growth is smoothly initiated with a mildly acidic to slightly alkaline pH range [1]. M. oleifera is considered an indigenous plant in the Southern region of Asia, its natural distribution spans the Himalayan foothills, from northeastern Pakistan through northwestern Bengal in India, and extends into the plains of Nepal. However, its cultivation has extended beyond its native regions to encompass various parts of Africa, Asia, and Europe [2]. This plant has been used as a traditional medicine in various countries for centuries to cure diseases. M. oleifera is considered a super food due to its exceptional nutritional profile. It is a rich source of natural antioxidants, minerals, vitamins, and proteins, along with various other bioactive compounds [3-4].
Every part of this plant (leaves, pods, bark, sap, flowers, seeds, seed oil, and roots) has been used to treat diseases as an ethnomedicine [5]. M. oleifera's bioactive compounds are mostly found in leaves [6]. Many researchers have reported that M. oleifera leaves contain bioactive compounds with antibiotic, antifungal, anti-inflammatory, antitumor, anticancer, antihyperglycemic, antifibrotic, and antioxidant properties [7]. These pharmacological activities are related to the phytochemical content of M. oleifera leaf extract. Phytochemical test results have documented that M. oleifera leaf extract contains secondary metabolite compounds including alkaloids, phenols, hydroquinone, flavonoids, steroids, triterpenoids, tannins, and saponins [8-10]. Polyphenolic compounds have been found with high concentrations in M. oleifera leaves. The concentration of these compounds ranged from 2090 to 12,200 mgGAE/100 g of dry weight, and 1600 to 3400 mgTAE/100 g of DW in some cases, which indicate that M. oleifera is an excellent source of polyphenols [11].
Environmental heterogeneity significantly modulates the synthesis and accumulation of primary and secondary metabolites in plant species. The biological activity of plants is influenced by several factors associated with its geographic origin, climatic conditions, and edaphic (soil-related) characteristics [12]. Phytochemicals accumulations alter in plants in response to abiotic and biotic conditions in pathogens attack. Defense response of plants against abiotic stress or disease attacks caused by microorganisms. In these conditions, plants synthesize various antioxidant chemical compounds to protect themselves from stress-induced oxidative stress, while antimicrobial compounds are used as defense compound against pathogen attacks [13].
Particularly in medicinal plants, environmental factors influence on metabolic pathways, thereby changes the production of bioactive compounds. This phenomenon arises from the inherent chemical complexity of herbal medicines. The type and quantity of secondary metabolites along with their associated biological effects, exhibit a dynamic response to environmental fluctuations [14]. In this consequence, it is imperative to investigate the intraspecific metabolite variation, their significant values in multiple beneficial plant species, and effects of diverse geographical regions, which are yet to be explored. Therefore, in this study we analyzed the phytochemical content of M. oleifera plants originating from two regions with different agroclimatic conditions based on altitude and temperature in South Sulawesi, Indonesia. Initially, we identified a series of metabolites those accumulation varied in response to divergence of geographic locations and agroclimatic conditions.
MATERIALS AND METHODS
Sampling
Total two locations were considered for M. oleifera plants sampling. Location 1 was Enrekang Regency (3°17'32"S, 119°46'16''E,) with high altitude and low temperature (coded with M1), and Location 2 was Jenneponto Regency (5°40'17"S, 119°48'19''E,) with low altitude and high temperature (coded with M2), in South Sulawesi Province, Indonesia. Bright green leaves from the 3-5 stalks below the shoot of M. oleifera were collected for metabolite analyses.
Compound extraction
M. oleifera leaves were picked from the leaf stalks and macerated with 96% methanol solvent (Fulltime, China) for three days. The leaf methanol extract was evaporated using a rotary evaporator and concentrated extract was collected further analyses.
Phytochemical screening
Phytochemicals were screened from M. oleifera leaf extract according to the method described previously [15]. Briefly, 2 mL of hydrochloric acid was mixed with 2 mL of extract. Next, put a couple of drops of Mayer's reagent. The green hue or white solid indicates the presence of alkaloids. A total 0.5 mL of the extract, 2 mL of chloroform (Fulltime, China), and concentrated sulphuric acid (Merck, Germany) were homogenized. The solution provided reddish-brown color formation at the interface shows the existence of Terpenoids. A total 0.5 mL of the extract was mixed with 2 mL of chloroform and 1 mL of sulphuric acid (H2SO4) (Merck, Germany). The development of a coppery brown circle at the interface suggests the existence of steroids. A total 1 mL of a solution containing 5% FeCl3 (Merck, Germany) was mixed with 1 mL of the extract. The development of a dark blue or greenish-black hue suggests that tannins are present. A total 2 mL of extract were mixed with 2 mL of distilled water and shaken in a graduated cylinder for a period of 15 minutes lengthwise. The existence of saponins was detected by the creation of a 1 cm foam layer.
A total 2 mL of extract were mixed with 1 mL of 2 normal sodium hydroxide (NaOH) (Merck, Germany). Yellow color formation indicated the presence of flavonoids in solution mixture. A total 2 mL of distilled water followed by a few drops of 10 % ferric chloride (Merck, Germany) was added to 1 mL of the extract. The formation of blue or green color indicated the presence of phenols. A total 1 mL of a 10 percent solution of sodium hydroxide (Merck, Germany) was combined with 1 mL of extract. The formation of a yellow hue indicated the coumarins are present in extract. A total of 1 mL of concentrated H2SO4 (Merck, Germany) was mixed with 1 mL of extract. The occurrence of a red hue signifies that quinones were present. A total 2 mL of extract was mixed with 3 mL of chloroform (Fulltime, China) and a 10% ammonium (Merck, Germany) solution. The formation of a pink hue demonstrates the existence of glycosides.
Total phenol and flavonoid content analysis
Total phenol content in leaf extract was measured according to the method of Aryal et al. (2019) using the Folin-Ciocalteu reaction (Merck, Germany). A total 200 µL of extract (1 mg/mL) with 200 µL of 50% Folin Ciocalteau reagent was vortexed for 1 minute. Following that, 4 mL of 2% sodium carbonate (Merck, Germany) was added to the mixture, and incubated at room temperature with dark condition for 30 minutes. The absorbance was measured at 750 nm with a UV-Vis spectrophotometer. The experiment was repeated three times. The calibration line was produced using gallic acid as a reference solution at concentrations ranging from 6.25-200 µg/mL. The total phenolic content was reported as gallic acid equivalents (mg GA/gram extract).
Total flavonoid content in extract was determined following the reference used previously [17], where quercetin was used as standard. A total of 10 mg of extract was diluted in 10 mL of distilled water to create a 1000 ppm solution. A total 500 µl of extract solution with 2 mL of distilled water and 0.15 mL of NaNO2 (Merck, Germany) was incubated at room temperature for 6 minutes. A total 0.15 mL of AlCl3 (Merck, Germany) was added to the solution mixture and homogenized then incubated for 6 min. A total 2 mL of 1N NaOH (Merck, Germany) was added to the extract solution and incubated for 15 minutes. The absorbance was measured at 352 nm. The total flavonoid content was reported as quercetin equivalents (mg of QE/g extract).
Antioxidant analysis using the DPPH method
Antioxidant activity was determined by the free radical scavenging method using DPPH (1,1-Diphenyl-2-picrylhydrazyl) (Merck, Germany) and vitamin C (Merck, Germany) as a control. A stock solution of vitamin C was prepared by dissolving 10 mg of ascorbic acid reference standard in methanol to a final volume of 10 mL, yielding a concentration of 1000 ppm. Subsequent serial dilutions were performed to generate a calibration curve encompassing five concentrations: 3 ppm, 6 ppm, 9 ppm, 12 ppm, and 15 ppm. The sample solution was prepared by dissolving 10 mg extract with methanol in a 10 mL volumetric flask (1000 ppm). The sample solution was diluted into four concentrations (5 ppm, 10 ppm, 25 ppm, 50 ppm, and 100 ppm). The test solution and positive control were added to each test tube, followed by 1 mL of DPPH solution, 5 mL of pro-analysis methanol, and homogenization with a blank solution. The test solution and positive control were incubated at 37 °C for 30 minutes, and the absorbance from each solution was measured at 517 nm using a UV-VIS spectrophotometer. The antioxidant activity of samples was determined and is then calculated using the following formula:
A linear regression equation was generated by inserting the test solution's concentration value as the x-axis and the percentage resistance as the y-axis. The IC50 is derived by inserting a resistance of 50% into the linear regression equation. Following analysis, very strong antioxidants was considered when the IC50 value was < 50 ppm, strong when the IC50 value was 50-100 ppm, moderate when the IC50 value was 101-150 ppm, and weak when the IC50 value was> 150 ppm [18].
Identification of functional groups using FTIR
A total of 1.5–2.0 g of sample extract was mixed gently with 200 mg of solid KBr and ground to make pellets. A standard device was used to pellet under vacuum and pressure (75 kN cm-2) for 2-3 minutes. This pellet was then used for functional group spectral analysis with FTIR (Thermo Fisher Scientific, U.S). The spectral resolution was 4 cm-1 with a scanning range of 400-4000 cm-1.
Identification of compounds by GC-MS analysis
Secondary metabolites were Identified using GC-MS (Shimadzu, Japan). The extract solution was injected into the GC-MS. The injection temperature was set at 250 °C. The GC-MS column temperature was set at 70 °C and held for 1 minute, then increased to 7 °C at a speed of 7 °C /minute, then increased again at a speed of 7°C /minute until the water temperature was 250 °C and incubated for 5 minutes.
Prediction of antibacterial and antioxidant compounds of M. oleifera extract using molecular dockings
Antibacterial and antioxidant compounds in M. oleifera leaf methanol extract was determined using molecular docking. The ligands used were 3 dominant compounds from both samples, the control ligands were ascorbic acid and penilisin. The receptors used for antioxidants are Chitocrome oxidase (PDB ID: 1nmL) and β-Ketoacyl-acyl carrier protein synthase III (FabH) (PDB Code: 1ebl). Ligand was prepared using the PyRx software suite, followed by energy minimization was done to optimize the conformational landscape and reduced the activation energy barrier. Ligands are converted into file format.pdbqt. Saved again in .pdb form. The 2D structure of red onion (Allium cepa) which was used as a ligand, while the control ligand was obtained from pubChem (http://pubchem.ncbi.nlm.nih.gov). Protein preparation was carried out with PyMol. Protein files have been downloaded from the Protein Data Bank (http://www.rcsb.org/pdb/), with non-protein molecules removed. Following purification, the protein structure was saved in the Protein Data Bank (.pdb) file format for subsequent docking simulations. AutoDock Vina, integrated within the PyRx software suite, was employed for the molecular docking studies. The docking protocol was followed, and the importation of the prepared ligand was completed and macromolecular target (receptor) was set using the software. A grid box encompassing the putative active site(s) of the protein was then meticulously defined. Subsequently, the docking simulation was initiated, and prompting of software was done to calculate the intermolecular interaction energies (scoring values) between the ligand and receptor upon user command. The docking results in the navigator are then saved as PDB to maintain proper detection of the ligand and receptor complex. Interactions between target ligands and proteins were visualized and analyzed using the Discovery Studio 2024 program by inputting docked ligand and receptor files from Autodock Vina.
RESULTS
Phytochemical analysis
Phytochemical content in M. oleifera leaves was obtained from two different locations and showed positive results. M1 code for location 1 (low temperature and high altitude) and M2 code for location 2 (hot temperature and low altitude). Both samples showed positive results in all compound tests carried out (Table 1). These results also revealed that in both environmental conditions the plant, M. oleifera produces all the tested compounds necessary for plant growth. Although the two samples contained the same types of phytochemical compounds, but the concentrations of phenols and flavonoids were different. Table 2 shows that the total phenols and flavonoids in M. oleifera leaves in M1 were significantly higher than in M2.
Table 1. Phytochemical analysis of M. oleifera leaves from different geographical and agroclimatic environments.
Table 2. Total phenol and flavonoid content of methanol extract of M. oleifera leaves from different geographical and agroclimatic environments.
Antioxidant activity
The results of the antioxidant activity of M. oleifera leaf samples from different agroclimatic conditions show that both M. oleifera leaves have high antioxidant activity with a high concentration level of IC50 (IC50 <50 ppm), and the level was higher than the antioxidant activity of vitamin C. The IC50 concentration and the antioxidant capacity were inversely proportionate, while sample M1 was identified as having the highest antioxidant capacity compared with M2 and vitamin C as positive control. The IC50 was 3.18 ppm, 10.49 ppm, 36.16 ppm, respectively (Figure 1).
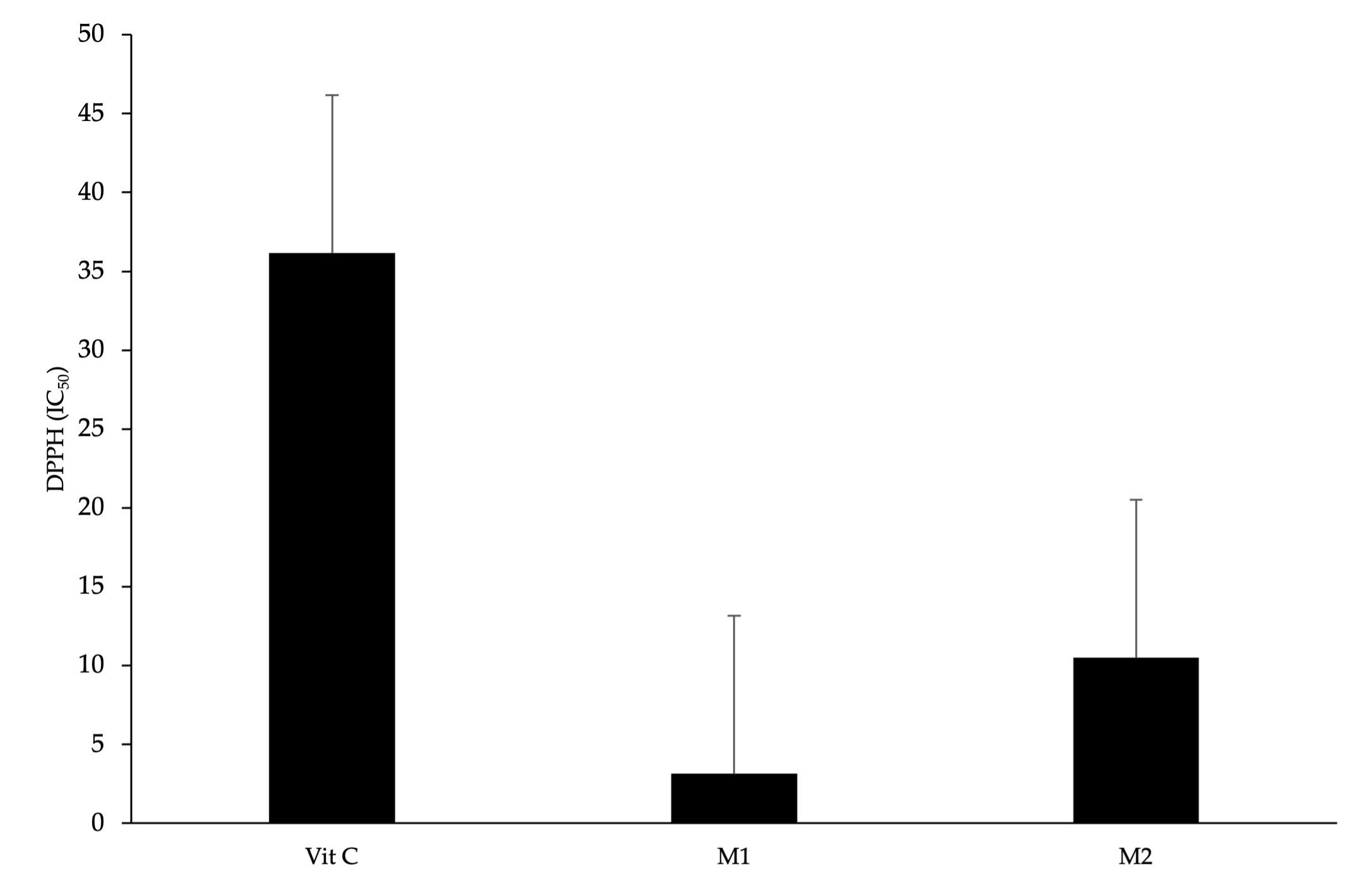
Identification of bioactive compounds by FTIR and GC-MS
Bioactive compounds were detected by phytochemical test using FTIR analysis of both M1 and M2 extracts in the area 4000- 400 cm-1. Figure 2 shows the FTIR spectra results of the two extracts show different spectral patterns indicating that the two samples contained qualitatively different compounds. Sample M1 shows a varied fingerprint area compared to sample M2, which suggests that the compounds produced in sample 1 were more diverged. This reveals an environmental influence on the compound content of M. oleifera leaves from different locations. However, both extract samples showed the presence of the OH group at wave number 3413.96 cm-1 in sample M1 and 3451.78 cm-1 in sample M2. The region 3400–3200 cm−1 shows the polymer hydroxyl groups (O–H), and H-bond stretching, which are the characteristics of polyphenolic compounds [19]. The existence of this wave number indicates that both samples contain phenolic compounds but at different concentrations which are indicated by different absorption intensities. These results were obtained by measuring the total levels of phenolics, flavonoids, and antioxidant activity of the two samples.
The acquisition of bioactive compounds identified in samples M1 and M2 using GC-MS. The dominant compounds found in the M1 sample including Levoglucosan (13.6%), Benzeneacetonitrile 4-hydroxy (12.24%), and 1,3,4,5-tetrahydroxycyclohexanecarboxylic acid (11.61%), while the dominant compounds found in sample M2 were 9,12,15-Octadecatrienoic acid, (Z,Z,Z) (16.38%), Quinic acid (12.63% ), and n-hexadecanoic acid (11.22%) (Figure 3).
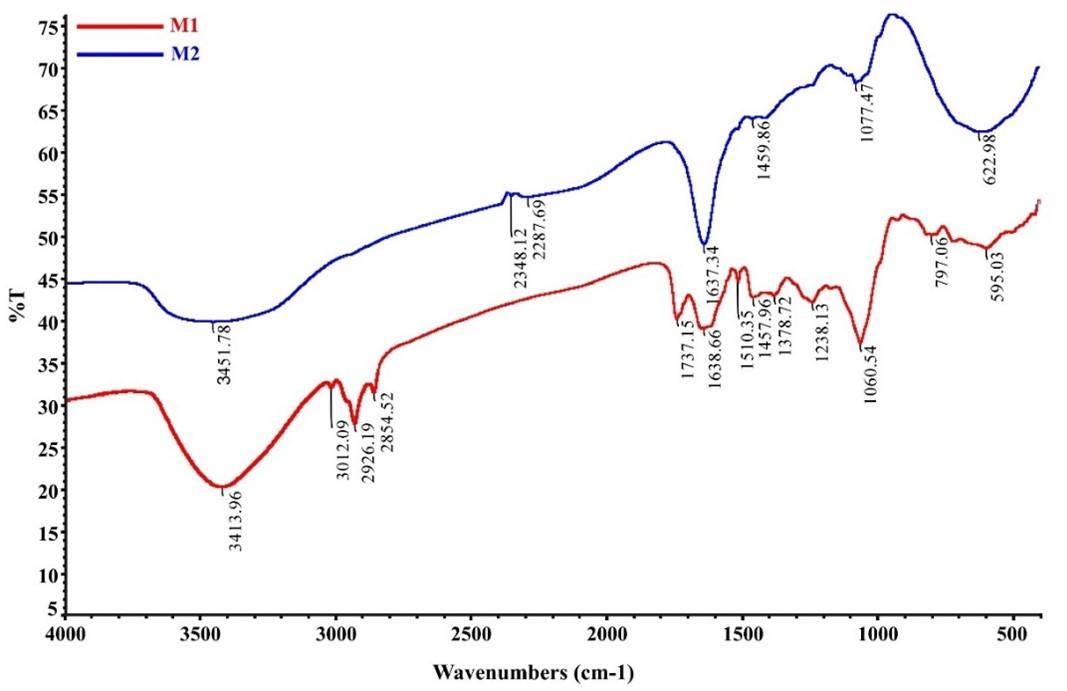
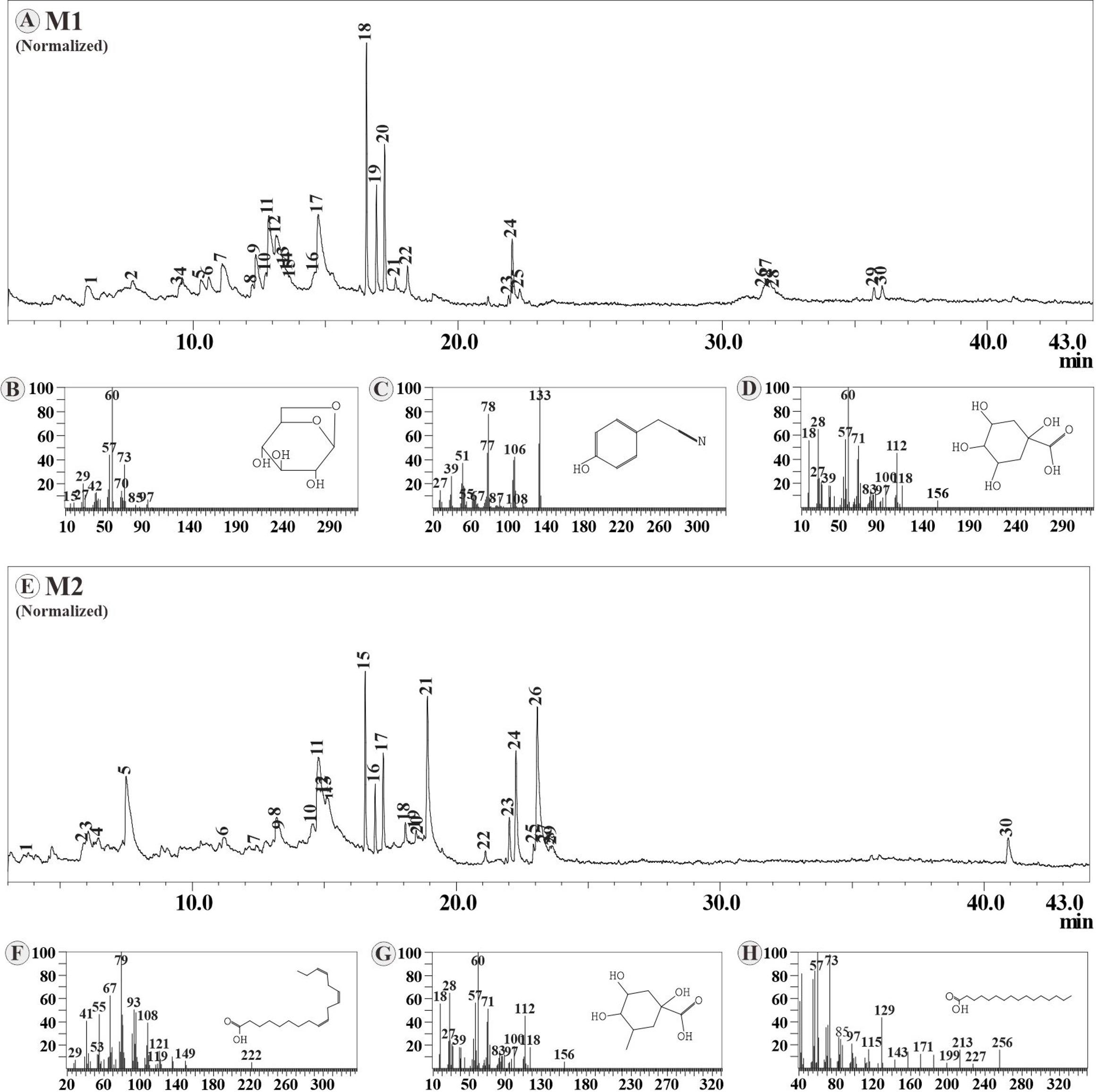
In silico antioxidant activity by molecular docking
The dominant compound in each sample as an antioxidant, molecular docking was conducted between the ligand compound and Cytochrome c peroxidase (PDB code: 1nmL) with ascorbic acid as ligand control. 1,3,4,5-Tetrahydroxycyclohexanecarboxylic acid compounds in M1 exhibited very promising binding scores (-6,9 kcal/mol) compared to the binding score of ascorbic acid (-6.6 kcal/mol), while Benzeneacetonitrile,4-hydroxy exhibited same binding score to ascorbic acid. Levoglucosan exhibited a higher binding score but was still in the tolerance range (-6,2 kcal/mol). These compounds stabilized inside the binding pocket of Cytochrome c peroxidase by binding with crucial amino acids (Tabel 3).
All dominant compounds in M2 highly exhibited, which were very promising antioxidants compared to ascorbic acid (control). The binding score of Octadecatrienoic acid was -7.3 kcal/mol, Quinic acid was -7.0 kcal/mol and n-hexadecanoic acid was -7.0 kcal/mol. These compounds stabilized inside the binding pocket of Cytochrome c peroxidase by binding with crucial amino acids (Table 4).
Ascorbic acid ligand control to active site Cytochrome c peroxidase via bound with six crucial amino acids (Ser24, Ser80, Asp62, Thr82, Phe260, and Asn79), Levoglucosan bound via Thr82, Ser80 and Thr256 amino acid. 1,3,4,5-tetrahydroxycyclohexanecarboxylic acid bound via Asn79, Phe260, Ser248, Thr82 and Asp62 amino acid, and Benzeneacetonitrile,4-hydroxy bound via Arg246, Phe93, Phe260 and Ser248 amino acid. Octadecatrienoic acid bound to active site Cytochrome via Thr256, Pro258, Pro81, Phe93, His261, Phe93, His261, Phe245, Phe260, met275, Val235, Ala247 and Tyr212 amino acid. Quinic acid bound via Pro81, Thr82, Asp62, Thr256, and Ser248 amino acid. n-Hexadecanoic acid bound via Asp62, Thr256, Thr82, Phe260, Ile253, Tyr259, Leu250, Ala271, Met275, Val272, Leu296, Ile292, Cys197. The 2D ligand-receptor is shown in Figure 4.
Table 3. Binding affinity score dominant compounds in M1 - cytochrome c peroxidase.
Table 4. Binding affinity score of dominant compounds in M2 - cytochrome c peroxidase.
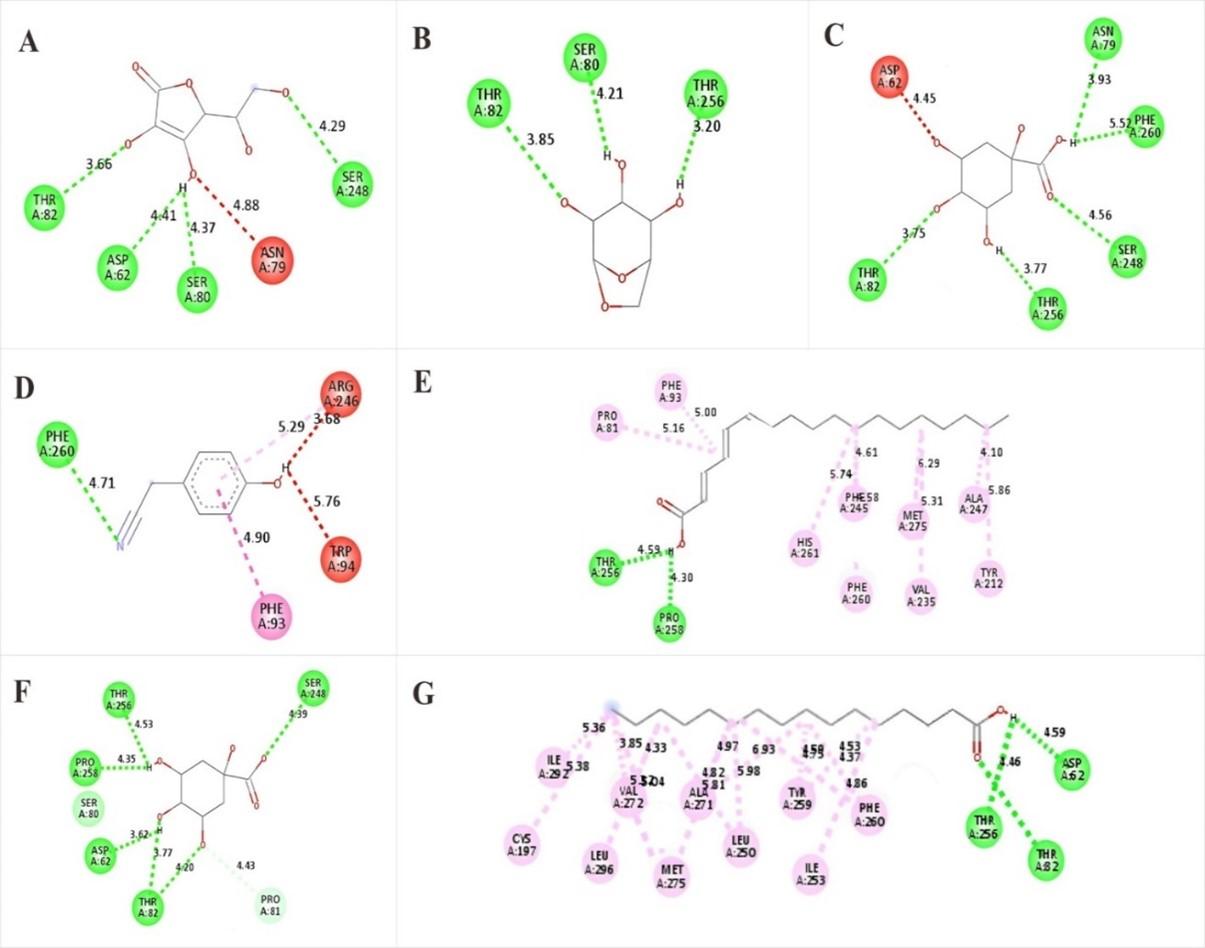
DISCUSSION
This study provided numerous phytochemicals, including alkaloids, tannins, saponins, flavonoids, and glycosides in M. oleifera leaves methanol extract. Methanol was used due to its capability of attracting more compounds. Previous studies have documented that methanol solvent can attach more phytochemical compounds in the case of even M. oleifera leaf extract [20]. Alcohols, particularly ethanol and methanol, are frequently employed as solvents in phytochemical extractions due to their ability to dissolve a broad spectrum of polar and moderately polar secondary metabolites. Methanol often exhibits superior extraction efficiency compared to ethanol. This phenomenon can be attributed, in part, to the polarity and solvating properties of methanol, which facilitate the disruption of plant cell walls and enhance the mass transfer of target analytes into the solvent [21]. The principle is that a compound will dissolve in a solvent that has similar properties, and the similarity in polarity to the solvent determines the yield of the compound [22].
Positive detection of compounds proves that all those compounds are needed for the plant. Phytochemicals produced by plants are used for plant growth, physiological processes, development, and protection [23, 24]. Although the alkaloids regulate multiple functions, including nitrogen storage, defense against predators, and growth regulation. Some alkaloids have similar structures known as plant growth regulators [25]. Glycosides play a multifaceted role in plant growth and development. They serve not only as energy and carbon sources but also as important regulators interacting with various signaling molecules, including phytohormones [26]. Previous reports have suggested that terpenoids provide their flavor to plants; tannins and quinones are responsible for the pigmentation of the plant [27-29]. Quinones are electron carriers that play a function in photosynthesis. Quinones' antioxidant action improves overall health [29, 30]. Coumarins are involved in many things for plants, such as growth hormones and plant growth regulators, controlling photosynthesis, respiration, and infection resistance [31]. Furthermore, glycosides contribute significantly to plant biochemistry and physiology by functioning as antioxidants, enzyme inhibitors, and precursors to bioactive compounds with potential defense mechanisms [32]. Interestingly, saponins, another class of secondary metabolites, have been demonstrated to promote both seed germination and subsequent plant growth rate [33].
The phenols and flavonoids help to adapt plants to environmental conditions. Phenols are linked to improved plant adaptability to stressful circumstances [34]. Flavonoids are present in all plant tissues, although the quantity varies mostly on the species and habitat. The composition, abundance, and bioactivity of plant secondary metabolites are demonstrably influenced by a complex interplay of environmental factors. These factors encompass habitat type, prevailing abiotic factors (e.g., temperature, light, water availability), seasonal variations, and the presence or absence of stressful stimuli [35][36]. This intricate interplay of bioactive metabolite levels not only varies between plant species but also among genotypes that are exposed to differing environmental variables such as soil composition, water availability, and temperature regimes [37].
The environmental conditions for sample M1 with highlands and low temperatures (1335 meters above sea level with temperatures ranging between 21-23°C). However, these conditions have had a high environmental stress effect on M. oleifera plants. The environmental temperature was exceeded the level that induced the stress effect on M. oleifera plants. The optimum range of M. oleifera growth is in the temperature range of 25 - 35°C and the altitude should be below 600 m [38].
Plants are sessile organisms that respond to stressful conditions by changing the gene expression patterns that regulate the synthesis of metabolites involved in the interaction of plants and the environment [34]. The M1 environmental condition provides stressful conditions for the growth of M. oleifera so that higher levels of phenol and flavonoids are produced compared to M. oleifera at M2 condition. This might be related to the plant's defense systems against the greater solar radiation and ultraviolet light, which indicate the high altitude habit [39]. Intense irradiance, particularly UV light, triggers the production of reactive oxygen species (ROS) within plant tissues. The ROS acts as a signaling molecule, stimulating the biosynthesis of flavonoids, notably anthocyanins, which function as protective pigments [40]. The number of phenolic compounds, phenols, and flavonoids in plants are involved in regulating plant growth and metabolic pathways in response to stress conditions. Under environmental stress conditions at M1, ROS increase and disrupt biochemical and physiological activities, thereby endangering plants [41]. In conditions of oxidative stress, plants produce enzymatic and non-enzymatic antioxidants. Phenols and flavonoids are enzymatic antioxidants that directly and indirectly protect plants from oxidative damage, also been proven that geographical and climate variations impact total phenol and flavonoid Ecalyptus camaldulensis leaves [42-44].
Phenolic compounds are at the vanguard of several scientific areas among components of phytochemicals found in plants. This significance is mostly attributed to polyphenols' ability to prevent a wide range of illnesses, the most common of which are reactive oxygen species, inflammation, diabetes, cardiovascular disease, and others. Flavonoids are hydrolyzable and condensed tannins, and phenolic acids are among its constituents. These substances contain antioxidant properties as hydrogen donors, reducing agents, and free radical scavengers account for their effectiveness [45]. Phenolic substances suppress free radicals by transferring hydrogen atoms from their hydroxyl groups. The chemical process between phenolic compounds and peroxyl radicals (ROO•) includes the transfer of hydrogen cations from phenol to the radical, generating a transition state HO bond with one electron [46]. Flavonoids exert their antioxidant properties through a multifaceted approach, including direct free radical scavenging. In this process, flavonoids donate electrons to neutralize ROS, resulting in the formation of more stable flavonoid radicals and a concomitant reduction in ROS-mediated cellular damage. Flavonoids' hydroxyl groups are very reactive, rendering the radicals inactive. Some flavonoids can directly scavenge superoxide, whereas others scavenge a highly reactive oxygen-derived radical termed peroxynitrite [47].
The antioxidant activity of both samples was in line with the total phenol and flavonoid content. Sample M1 had higher levels of total phenols, flavonoids, and antioxidant activity than sample M2. These variations occurred due to the variation of geographical and agroclimatic conditions namely temperature, humidity, and altitude, which influence the phytochemical content and antioxidant activity of M. oleifera leaves. Sample M1 obtained from location 1 grew not in optimum conditions, thus stress was not induced in plants. However, this environmental stress results in high phytochemical content and antioxidant activity, which plays a role in the adaptation of M. oleifera to these unfavorable conditions. The bioactivity and mechanism of action of antioxidant compounds isolated from plant extracts exhibit a marked dependence on the plant's chemical composition, which in turn is significantly influenced by environmental factors. These factors modulate the synthesis of secondary metabolites within the plants. Plant habitat plays a crucial role in shaping the formation of these bioactive secondary metabolites. This current study shows that conditions like high humidity and low temperature at location M1 can increase plant extracts' antioxidant activity. A previous study has shown low temperatures induce antioxidant enzyme activity including CAT (catalase) and SOD (superoxide dismutase) is significantly higher than under normal conditions [48].
The research findings show that there is a correlation between altitude and IC50 in the two samples. The sample M1 contains an altitude of 1334 meters above sea level, while the M2 shows an altitude of 12 meters above sea level. Altitude as a peripheral environmental factor is correlated with several elements that make up plants. As altitude increases, IC50 decreases and antioxidant properties increase [49, 50]. The relationship between altitude and the impact of environmental stress showed the impact on active ingredients and the increase in free radical inhibitory potential and antioxidant capacity in tea plants, Azarum and Scrophularia striata plants [51, 52]. Based on this, it can be concluded that high altitudes involved in decreasing temperature and increasing soil moisture cause antioxidant compounds and enzyme activity. Therefore, the highest antioxidant activity was observed in sample M1. This study has been corroborated with a previous study [53]. However, the highest antioxidant activity of medicinal plants was obtained in plants in response to an altitude of 1370 meters above sea level, which is the same altitude range as M1.
FTIR analysis confirmed the presence of phytocompounds detected in phytochemistry screening in plants. The absorption at 1378.72 cm−1 indicates OH bending for phenol compounds. Phenolic C–O stretching was observed at ~ 1200 cm−1 in sample M1, this is stretching the typical nature of flavonoid C-rings. The presence of the C=O carbonyl group was also detected at wave number 1737.15 cm−1 in sample M1, C=CC aromatic ring at wave number 1510 -1450 cm−1. CH bending aromatic compound at 1638.66 cm−1. aliphatic CH was detected at wave numbers 2926.19, 2854.52 cm−1. In sample M2, it was detected absorption at 1459.86 cm−1 is a C = C aromatic double bond belonging to the flavonoid compound. CH bending aromatic compound was detected at absorption 1637.34 cm−1. The presence of the O=C=O strain was detected in absorption 2348.12 cm−1. Terpenoids were detected by absorption in the wave number range 1459.86 cm−1. The wave number 1490-1400 cm−1 is the absorption for OH RCO2H which indicates the presence of terpenoids. Terpenoids are compounds that have alcohol and carboxylic acid functional groups [54]. The observed natural products in M. oleifera leaves from the phytochemical analysis were confirmed. Those compounds have OH, C=O, CO, aliphatic, and aromatic carbons functional groups as displayed in the FTIR spectra [55].
Although phytochemistry screening and FTIR analysis showed the results for both samples. However, the GC-MS analysis revealed the variation of components in compounds. The differences in compounds from M. oleifera leaves due to the impact of the environment. In different environments, plants produce different compounds to adapt to certain conditions. The metabolite compounds produced are a way for plants to survive biotic and abiotic stress. Phytochemical content in plants is influenced by internal and external factors. Internal factors such as genes and external factors include light, temperature, humidity, pH, nutrient content in the soil, and altitude of the location [56]. Benzeneacetonitrile,4-hydroxy has been reported to be found in M. oleifera leaves [57]. 9,12,15-Octadecatrienoic acid and quinic acid as compounds identified from M. oleifera leaves [58]. Levoglucosan is a carbohydrate that has been reported in M. oleifera leaves [59].
According to in silico molecular docking, 1,3,4,5-Tetrahydroxycyclohexanecarboxylic acid in M1 and Octadecatrienoic acid in M2 promise as antioxidant natural sources. Those compounds had better binding scores compared to ascorbic acid (vitamin C) as ligand control. Ascorbic acid has been known well as a strong antioxidant. The better binding score indicated better activity than ascorbic acid. However, the result of total antioxidant activity measured by DPPH-potential of plant samples revealed the activity of extracts stronger than the control.
CONCLUSIONS
This study provides the impacts of geographical and agroclimatic locations on phytochemicals and the antioxidant activity of M. oleifera. Consequently, both studied samples from the two locations influence the accumulation of alkaloids, terpenoids, steroids, tannins, saponins, phenols, flavonoids, coumarins, quinones, and glycosides, which play a crucial role in plant growth and development in various environmental situations. However, the acquisition of phytochemicals may vary with their quantity and antioxidant and antibacterial potential in response to multiple environmental stress signals. Sample M1 from both high and low-temperature areas exhibited greater levels of total phenol and flavonoid content, as well as stronger antioxidant and antibacterial activity compared to sample M2. This outcome represents how M. oleifera adapted and defended itself in different environmental conditions. In the antibacterial test results, it was found that M1 has greater antibacterial effectiveness against both Gram-negative bacteria (Escherichia coli and Salmonella tipsy) and Gram-positive bacteria (Bacillus cereus and Staphylococcus aureus) compared to M2. The FTIR analysis revealed the presence of OH, C=O, CO, aliphatic, and aromatic carbon functional groups, signaling the existence of compounds identified through phytochemical analysis assays. Levoglucosan, Benzeneacetonitrile, 4-hydroxy, and 1,3,4,5-Tetrahydroxycyclohexanecarboxylic acid were the main compounds in sample M1 according to GC-MS analysis, whereas sample M2 contained 9,12,15-Octadecatrienoic acid, (Z,Z,Z), Quinic acid, and n-Hexadecanoic acid as the dominant compound. The antioxidant potential of the identified compound was confirmed through molecular docking. However, the above findings from this current study suggest the impacts of geographic location and agroclimatic conditions on metabolites accumulations in M. oleifera. This study might be useful to serve as a guide for choosing active compound sources for different pharmacological interests.
ACKNOWLEDGEMENTS
There is no external funding for this research. The authors would like to thank Hasyimuddin for the support in sample collection at Enrekang Regency, the team of Microbiology Lab UIN Alauddin Makassar for supporting in preparing the research, and Syarif Hidayat Amrullah for supporting in turnitin checking.
AUTHOR CONTRIBUTIONS
ES, AA, AK, ZD, and HK collaborated to execute the research plan, conduct practical research, and prepare the manuscript. DA, MM, and ZZ as a team for collecting data. RFA contributed to editing the manuscript. The finalized manuscript has been reviewed and approved by all authors.
CONFLICTS OF INTEREST
There is no conflict of interest among the authors.
References
- [1]Pareek A, Pant M, et al. Moringa oleifera: An Updated Comprehensive Review of Its Pharmacological Activities, Ethnomedicinal, Phytopharmaceutical Formulation, Clinical, Phytochemical, and Toxicological Aspects. Int J Mol Sci. 2023; 24: 2098.
- [2]Mahato DK, Kargwal R, et al. Ethnopharmacological properties and Nutraceutical potential of Moringa oleifera. Phytomedicine Plus. 2022; 2: 100168.
- [3]Gómez-Martínez M, Ascacio- Valdés JA, et al. Location and tissue effects on phytochemical composition and in vitro antioxidant activity of Moringa oleifera. Ind Crops Prod. 2020; 151: 112439. DOI:
- [4]Hossain MA, Disha NK, et al. Determination of Antioxidant Activity and Total Tannin from Drumstick (M. oleifera Lam.) Leaves Using Different Solvent Extraction Methods. Turkish J Agric-Food Sci Technol. 2020; 8: 2749–2755.
- [5]Stohs SJ and Hartman MJ. Review of the Safety and Efficacy of Moringa oleifera. Phytother Res. 2015; 29: 796–804.
- [6]Rani NZA, Husain K, et al. Moringa genus: A review of phytochemistry and pharmacology. Front Pharmacol. 2018; 9: 1–26.
- [7]Kashyap P, Kumar S, et al. Recent Advances in Drumstick (Moringa oleifera) Leaves Bioactive Compounds: Composition, Health Benefits, Bioaccessibility, and Dietary Applications.” Antioxidants (Basel, Switzerland). 2022; 11: 402.
- [8]Khalid S, Arshad M, et al. Nutritional and phytochemical screening of M. oleifera leaf powder in aqueous and ethanol extract. Int J Food Prop. 2023; 26: 2338–2348. DOI: 10.1080/10942912.2023.2246685.
- [9]Sankhalkar S and Vernekar V. Quantitative and Qualitative Analysis of Phenolic and Flavonoid Content in M. oleifera Lam and Ocimum tenuiflorum L. Pharmacognosy Res. 2016; 8: 16–21.
- [10]Fachriyah E, Kusrini D, et al. Phytochemical Test, Determination of Total Phenol, Total Flavonoids and Antioxidant Activity of Ethanol Extract of Moringa Leaves (M. oleifera Lam). J Kim Sains dan Apl. 2020; 23: 290–294.
- [11]Azlan UK, Mediani A, et al. A Comprehensive Review with Updated Future Perspectives on the Ethnomedicinal and Pharmacological Aspects of Moringa oleifera. Molecules. 2022; 27: 5765.
- [12]Xu W, Cheng Y, et al. Effects of geographical location and environmental factors on metabolite content and immune activity of Echinacea purpurea in China based on metabolomics analysis. Ind Crops Prod. 2022; 189: 115782. DOI: https://doi.org/10.1016/j.indcrop.2022.115782.
- [13]Mohiuddin AK. Journal of Pharmacology & Clinical Research AK Mohiuddin. Impact of Various Environmental Factors on Secondary Metabolism of Medicinal Plants. J Pharmacol Clin Res. 2019; 7: 555704.
- [14]Yang L, Wen KS, et al. Response of plant secondary metabolites to environmental factors. Molecules. 2018; 23: 1–26.
- [15]Mane RS and Vedamurthy B. Mycochemical analysis and anticancer activity of endophytic Aspergillus nomius against MCF7 human breast cancer cell line. Int J Pharma Bio Sci. 2020; 11: 1–6.
- [16]Aryal S, Baniya MK, et al. Total Phenolic content, Flavonoid content and antioxidant potential of wild vegetables from western Nepal. Plants. 2019; 8: 96.
- [17]Sulastri E, Zubair MS, et al. Total phenolic, total flavonoid, quercetin content and antioxidant activity of standardized extract of M. oleifera leaf from regions with different elevation. Pharmacogn J. 2018; 10: S104–S108, 2018.
- [18]Sukweenadhi J, Setiawan F, et al. Antioxidant activity screening of seven Indonesian herbal extract. Biodiversitas. 2020; 21: 2062–2067.
- [19]Wongsa P, Phatikulrungsun P, et al. FT-IR characteristics, phenolic profiles and inhibitory potential against digestive enzymes of 25 herbal infusions. Sci Rep. 2022; 12: 1–11.
- [20]Bhalla N, Ingle N, et al. Phytochemical analysis of M. oleifera leaves extracts by GC-MS and free radical scavenging potency for industrial applications. Saudi J Biol Sci. 2021; 28: 6915–6928.
- [21]Zhang QW, Lin LG, et al. Techniques for extraction and isolation of natural products: A comprehensive review. Chinese Med (United Kingdom). 2018; 13: 1–26.
- [22]Herawati E, Ramashan R, et al. Phytochemical screening and antioxidant activity of wild mushrooms growing in tropical regions. Biodiversitas. 2021; 22: 4716–4721.
- [23]Kumar A, Kumar PN, et al. Major Phytochemicals: Recent Advances in Health Benefits and Extraction Method. Molecules. 2023; 28: 887.
- [24]Guan R, Van LQ, et al. A review of dietary phytochemicals and their relation to oxidative stress and human diseases. Chemosphere. 2021; 271: 129499.
- [25]Ali AH, Abdelrahman M, et al. Alkaloid Role in Plant Defense Response to Growth and Stress. Bioactive Molecules in Plant Defense: Signaling in Growth and Stress. 2019: 145–158.
- [26]Jeandet P, Formela-Luboińska M, et al. The Role of Sugars in Plant Responses to Stress and Their Regulatory Function during Development. International journal of molecular sciences. 2022; 23: 5161.
- [27]Kregiel D, Berlowska J, et al. Saponin-Based, Biological-Active Surfactants from Plants. Application and Characterization of Surfactants. 2017; 6: 184-205.
- [28]Huang Y, Xie FJ, et al. Research progress in biosynthesis and regulation of plant terpenoids. Biotechnol Biotechnol Equip. 2021; 35: 1799–1808.
- [29]Roba K. The Role of Terpene : Secondary Metabolite. Nat Prod Chem Res. 2021; 9: 1–11.
- [30]El-Najjar N, Gali-Muhtasib H, et al. The chemical and biological activities of quinones: overview and implications in analytical detection. Phytochem Rev. 2011; 10: 353–370.
- [31]Robe K, Izquierdo E, et al. The Coumarins: Secondary Metabolites Playing a Primary Role in Plant Nutrition and Health. Trends Plant Sci. 2021; 26: 248–259. DOI:
- [32]Chattha FA, Mehr‐un‐Nisa, et al. Coumarin‐Based Heteroaromatics as Plant Growth Regulators. In Plant Growth: IntechOpen, 2016.
- [33]Durán AG, Calle JM, et al. Steroidal Saponins with Plant Growth Stimulation Effects; Yucca schidigera as a Commercial Source. Plants. 2022; 11.
- [34]Šamec D, Karalija E, et al. The Role of Polyphenols in Abiotic Stress Response: The Influence of Molecular Structure. Plants (Basel, Switzerland). 2021; 10: 118.
- [35]Stanković MS, Petrović M, et al. Screening inland halophytes from the central Balkan for their antioxidant activity in relation to total phenolic compounds and flavonoids: Are there any prospective medicinal plants?. J Arid Environ. 2015; 120: 26–32.
- [36]Stawarczyk K, Chrupek A, et al. Insight into the Way the Content of Biologically Active Compounds in Meadowsweet Inflorescences (Filipendula ulmaria (L.) Maxim.) Is Shaped by Phytosociological Habitats. Molecules. 2021; 26: 5172.
- [37]Goławska S, Łukasik I, et al. Flavonoids and Phenolic Acids Content in Cultivation and Wild Collection of European Cranberry Bush Viburnum opulus L. Molecules. 2023; 28: 2285.
- [38]Trigo C, Castelló ML, et al. Moringa oleifera: An Unknown Crop in Developed Countries with Great Potential for Industry and Adapted to Climate Change. Foods (Basel, Switzerland). 2020; 10: 31.
- [39]Zoratti L, Jaakola L, et al. Modification of Sunlight Radiation through Colored Photo-Selective Nets Affects Anthocyanin Profile in Vaccinium spp. Berries. PLoS One. 2015; 8: e0135935.
- [40]Dudareva L, Tarasenko V, et al. Involvement of Photoprotective Compounds of a Phenolic Nature in the Response of Arabidopsis thaliana Leaf Tissues to Low-Intensity Laser Radiation. Photochem. Photobiol. 2020; 96: 1243–1250.
- [41]Zafari S, Sharifi M, et al. Modulation of Pb-induced stress in Prosopis shoots through an interconnected network of signaling molecules, phenolic compounds and amino acids. Plant Physiol Biochem. 2016; 99: 11–20.
- [42]Shomali A, Das S, et al. Diverse Physiological Roles of Flavonoids in Plant Environmental Stress Responses and Tolerance. Plants (Basel, Switzerland). 2022; 1: 3158.
- [43]Kumar K, Debnath P, et al. An Overview of Plant Phenolics and Their Involvement in Abiotic Stress Tolerance. Stresses. 2023; 3: 570–585.
- [44]Rosendal E, Ouédraogo CJW, et al. Geographical variation in total phenolics, flavonoids and antioxidant activities of Eucalyptus camaldulensis leaves in Burkina Faso. African J Pure Appl Chem. 2020; 14: 51–59.
- [45]Thouri A, Chahdoura H, et al. Effect of solvents extraction on phytochemical components and biological activities of Tunisian date seeds (var. Korkobbi and Arechti). BMC Complement Altern Med. 2017; 17: 248. DOI: 10.1186/s12906-017-1751-y.
- [46]Santos-Sánchez NF, Salas-Coronado R, et al. Antioxidant Compounds and Their Antioxidant Mechanism. Antioxidants. 2019; 10: 129.
- [47]Panche AN, Diwan AD, et al. Flavonoids: An overview. J Nutr Sci. 2016; 5. DOI: 10.1017/jns.2016.41.
- [48]Soengas P, Rodríguez VM, et al. Effect of Temperature Stress on Antioxidant Defenses in Brassica oleracea. ACS omega. 2018; 3: 5237–5243.
- [49]Hashim AM, Alharbi BM, et al. Oxidative Stress Responses of Some Endemic Plants to High Altitudes by Intensifying Antioxidants and Secondary Metabolites Content. Plants (Basel, Switzerland). 2020; 9: 869.
- [50]Zeng Q, Dong G, et al. High Altitude Is Beneficial for Antioxidant Components and Sweetness Accumulation of Rabbiteye Blueberry. Front Plant Sci. 2020; 11: 1–9.
- [51]Martono B, Falah S, et al. Antioxidant Activities of GMB 7 Variety of Tea at Different Altitude. J Ind Beverage Crop. 2016; 3 53–60.
- [52]Pan L, Yang N, et al. Altitudinal Variation on Metabolites, Elements, and Antioxidant Activities of Medicinal Plant Asarum. Metabolites. 2023; 13: 1193.
- [53]Khan MN, Mobin M, et al. Impact of varying elevations on growth and activities of antioxidant enzymes of some medicinal plants of Saudi Arabia. Acta Ecol Sin. 2016; 36: 141–148.
- [54]Ndahawali S and Andayani S. Phytochemical Screening by FTIR Spectroscopic Analysis and Antibacterial Activity of Sesbania grandiflora Fraction Against Edwardsiella tarda. J Exp Life Sci. 2019; 9: 176-180.
- [55]Kurniawan YS. A Comparative Study on Phytochemical Screening and Antioxidant Activity of Aqueous Extract from Various Parts of Moringa oleifera. Indones J Nat Pigment. 2021;3: 43-47.
- [56]Jan R, Asaf S, et al. Plant secondary metabolite biosynthesis and transcriptional regulation in response to biotic and abiotic stress conditions. Agronomy. 2021; 11: 1–31.
- [57]Mathur M, Yadav S, et al. In vitro propagation and biosynthesis of steroidal sapogenins from various morphogenetic stages of M. oleifera Lam., and their antioxidant potential. Acta Physiol Plant. 2014; 36: 1749–1762.
- [58]Vats S and Gupta T. Evaluation of bioactive compounds and antioxidant potential of hydroethanolic extract of M. oleifera Lam. from Rajasthan, India. Physiol Mol Biol Plants. 2017; 23: 239–248.
- [59]Shi H, Yang E, et al. Dynamic changes in the chemical composition and metabolite profiles of drumstick (M. oleifera Lam.) leaf flour during fermentation. LWT. 2022; 155: 112973.