Immunomodulatory effect of aqueous extract of Termitomyces striatus mushroom on enterohemorrhagic Escherichia coli-infected mice
Abstract
Enterohemorrhagic Escherichia coli (EHEC) generates Shiga toxins that cause immunosuppression and diarrhea. Synthetic immunomodulatory drugs are associated with numerous severe effects, necessitating the need for substitute therapeutic agents. This study aimed to determine the immunomodulatory activity of aqueous extract of Termitomyces striatus in mice infected with EHEC. Mice in the positive control, negative control, and two extract-treated groups were administered orally with 200µL of 9×108 CFU/mL EHEC. From day 5, the positive control, negative control, and extract-treated mice were orally administered with levamisole (50mg/kg body weight (bw)), phosphate-buffered saline (vehicle), and aqueous extract of T. striatus (200 and 400mg/kg body weight), respectively. The control mice received the vehicle only. After the experiment, the mice were euthanized, blood was collected, and white blood cells (WBCs) and differentials were analyzed using a hematological analyzer. Immunoglobulin G (IgG), interleukin-4 (IL-4), and interferon-gamma (INF-γ) levels were also quantified using enzyme-linked immunosorbent assays. The extract significantly reduced elevated levels of WBCs, neutrophils, monocytes, eosinophils, and basophils, as well as significantly enhanced the levels of lymphocytes in EHEC-infected mice (p<0.05). Besides, the extract significantly lowered the levels of INF-γ while significantly increased the levels of IL-4 and IgG in EHEC-infected mice. Flavonoids, alkaloids, saponins, steroids, and phenolics are some of the phytochemicals that were detected in T. striatus. Together, these findings revealed that T. striatus extract modulated immune response in EHEC-challenged mice by lowering leucocyte counts and INF-γ, enhancing lymphocytes, IL-4, and IgG, suggesting that the extract could be developed as an immunomodulatory agent.
INTRODUCTION
The primary function of the immune system, which comprises innate and adaptive immunity is to protect the body against invading antigens and infectious agents [1]. The functions of the two immune systems are distinct from one another, but they collaborate closely. Innate immunity is the primary defense mechanism against an invasive pathogen. The host's antigen-independent defensive response is triggered by antigens either instantaneously or over several hours [2]. Innate immunity includes the skin and mucus membranes, phagocytic cells, edema-relate serum proteins, and cells that synthesize cytokines and inflammatory mediators [3]. Innate immunity is preceded by adaptive immunity. Adaptive immunity consists of cell-mediated response (T helper and cytotoxic T lymphocyte) and antibody response (B cell) [4].
The immune response is triggered by an antigen or microbial infection, which causes macrophages to bind to the antigen and communicate with T lymphocytes [5]. Escherichia coli is a gram-negative bacterium that is normally found in the gut (intestines) of healthy individuals. The majority of E. Coli strains are either safe or cause relatively brief diarrhea [6]. However, some of the strains, such as Enterohemorrhagic Escherichia coli (EHEC), can cause severe vomiting stomach cramps, and bloody diarrhea, thus triggering an immune response [7]. The EHEC serotype O157:H7 is responsible for hemolytic uremic syndrome and bloody diarrhea outbreaks globally [8].
The innate and adaptive immunity can be stimulated, suppressed, or modulated by synthetic or natural immunomodulators [9–11]. Immunomodulators are typically used in clinical medicine to treat infections, restore immunodeficiency, and reduce overactive immune responses. Immunomodulators include immunosuppressive and immunostimulatory agents. Immunostimulant medications such as levamisole are used to strengthen the immune system's baseline response, which is crucial for individuals with immune system deficiencies, thus increasing the body's resistance to infections. Immunosuppressive medications such as cyclosporine and glucocorticoids are used to suppress immune function, inhibit hypersensitivity, and address an organism's immune responses against its own healthy cells [12,13]. However, these medications are associated with a number of severe effects [14], necessitating the need for alternative agents.
Currently, immunomodulators derived from natural compounds, also known as phytochemicals, are preferred due to their minimal toxicity [15]. Flavonoids, quinones, terpenoids, alkaloids, phenolics, saponins, and vitamin C are the most prevalent phytochemicals that modulate immune response [16,17]. Termitomyces species are edible mushrooms that have been documented to possess beneficial medicinal properties [18,19]. Termitomyces striatus are used as immunostimulant agents by the communities residing in western Kenya [20]. Nevertheless, there is a lack of empirical evidence to validate these claims. This investigation aimed to assess the immunomodulatory activity of aqueous extract of T. striatus in EHEC-infected mice, as well as qualitative phytochemical analysis.
MATERIALS AND METHODS
Collection of medicinal mushrooms
Fresh T. striatus samples were collected from Kakamega County, Kenya. The samples were cleaned, shade-dried, and milled into a homogenous powder. A portion of the sample was botanically identified and authenticated by a taxonomist in the National Museum of Kenya and a voucher specimen was deposited in the herbarium (Reference: NMK/BOT 2017).
Extraction of mushroom sample
Powdered T. striatus weighing 300 g was macerated with 800 ml of distilled water and subsequently put in a water bath (60°C) for twenty minutes. After cooling to ambient temperature, the supernatant was decanted and centrifuged at 5400 rpm for ten minutes. To create a solid extract, the supernatant was filtered via Whatman® GF/C glass microfiber filter paper and then lyophilized at -15°C using freeze-drier.
Experimental animals
The Institute of Primate Research Animal Science Rodent Facility supplied the healthy male BALB/c mice, which were between 6 and 8 weeks old. The animals were housed in a polypropylene cage under 12 hours of darkness/light cycles at room temperature. They were fed mice pellets and supplied tap water ad libitum. Ethical clearance was sought from the Institute of Primate Research Institutional Scientific Ethic Review Committee (REFERENCE: ISERC/08/2020) in Kenya.
The mice were assigned 5 groups of four mice each: normal control, negative control, positive control, and two extract (dosages of 200 and 400mg/kg bw) groups. The dosages were arrived after conducting a pilot study. All the experimental groups were orally infected with EHEC ATTCC 51299 (sourced from the National Public Health Reference Laboratory, Kenyatta National Hospital, Nairobi, Kenya), except for the normal control group. The EHEC was confirmed using Shiga toxins test. Mice were administered with 200μl of approximately 3 McFarland standard 9 ×108 CFU/mL EHEC [21]. The bacteria were cultured overnight at 37°C in Mueller-Hinton Broth cultures, and suspensions were measured using turbidity equal to 3 McFarland standard 9 ×108 CFU/mL EHEC. The vehicle, phosphate-buffered saline (PBS), was administered to negative control mice. Levamisole (50mg/kg bw) was used as a reference drug in positive control mice. The extract, levamisole, and vehicle were administered orally using a gavage (gauge 21) at a volume of 0.1ml. The treatments were initiated from day 5 after mice were infected with the EHEC to day 21 of the experiment.
Collection of blood samples
On days 0, 5, 10, and 15, mice were euthanized using carbon IV oxide anesthesia and blood drawn through cardiac puncture. The blood was stored in EDTA (Ethylenediamine tetraacetic acid) tubes before white blood cell and differential counts analysis. On days 0, 7, 14, and 21, the mice were euthanized, and blood was collected, dispensed in plain blood collecting tubes, let to stand for thirty minutes, and subsequently centrifuged at 1000 rpm for ten minutes. The resulting serum was aspirated, stored in plain blood collecting tubes, and then refrigerated (-80°C).
Determination of leucocyte count
Leukocytes were analyzed using an automated hematology analyzer (Mindray BC 10 Hematological Analyzer, Zhejiang, China).
Determination of immunoglobulin G, interleukin-4 and interferon-γ
The levels of immunoglobulin G (IgG), interleukin-4 (IL-4), and interferon-gamma (INF-γ) in the serum samples were determined using the enzyme-linked immunosorbent assay (ELISA) technique. The optical densities were obtained using LT-4500 Microplate ELISA reader (Labtech International Ltd, East Sussex, TN22 1QQ, UK). The manufacturer's instructions were followed while analyzing the samples. The ELISA kits included ELISA Flex: Mouse immunoglobulin (IgG) ALP, ELISA Pro: Mouse IFN-γ and ELISA Pro: Mouse IL-4 (Mabtech AB, Nacka Strand, Sweden).
Qualitative phytochemical analysis
Several methods were used to determine the presence of selected phytocompounds.
Alkaloids
The extract (5 ml) was put in one molar hydrochloric acid and then heated in a steam bath. The resultant acidified solution was added with a few drops of Dragendroff's reagent. An indicator of alkaloids was the formation of a reddish-brown or orange precipitate [22].
Flavonoids
An equal amount (1 ml) of the extract and diluted NaOH. An intense/golden-yellow precipitate was an indicator of flavonoids [23].
Saponins
The extract (1 ml) was mixed with a few drops of NaHCO3 solution, followed by vigorous shaking, and then allowed to stand for 15-20 minutes. Saponins were indicated by the formation of foam exceeding 1 cm [24].
Steroids
The extract (250 mg) was mixed with 1 ml of chloroform in a test tube. A layer was formed by carefully adding 1.5 ml of strong sulfuric acid. The appearance of a reddish-brown color at the interface (steroidal ring) indicated steroids [25].
Phenolics
The extract (2 ml) was mixed with 1 ml of FeCl3 solution. The development of a blue-to-green coloring indicated the presence of phenolics [26].
Statistical data analysis
Raw data was tabulated in Microsoft Office (Excel spreadsheet) and then imported for analysis from the statistical software for data science (STATA) program version 18. The mean ± standard deviation was used to express descriptive statistics. Inferential statistic one-way ANOVA (analysis of variance) was employed to identify significant variations between different treatment groups. Bonferroni multiple comparisons were carried out in case of statistical differences using ANOVA. The level of significance was set at p < 0.05.
RESULTS
Effect of aqueous extract of T. striatus on WBC and differential counts in EHEC-infected mice
The WBC and differential counts in the extract-treated, levamisole-treated, negative control, and normal control mice were insignificant (p>0.05) on day 0 (Figure 1). On days 5, 10, and 15, the mice that were infected with EHEC revealed a substantial increase in WBC count compared to levels noted in normal (healthy) control mice (p<0.05; Figure 1). Upon therapy with T. striatus extract doses of 200 and 400mg/kg bw, the EHEC-infected mice showed a reduction in WBC count that statistically matched (p>0.05) those of the normal control mice on days 10 and 15. On day 15, the WBC counts in mice that were administered with extract at the two studied doses were significantly lower (p<0.05) relative to the count of EHEC-infected control mice (Figure 1).
The neutrophil levels were substantially greater in the EHEC-infected control mice relative to those in the normal control mice on days 5, 10, and 15 (p<0.05). On days 5, 10, and 15, the levels of neutrophils in extract-treated (200 and 400mg/kg bw) and levamisole-treated mice were considerably greater than those seen in healthy control mice (p<0.05). Besides, the neutrophil levels in EHEC-infected control mice were substantially higher than levels in the other treatment groups on days 5, 10, and 15 (p<0.05; Figure 1).
The lymphocyte counts in EHEC-infected control mice was significantly lower than those in extract-treated, levamisole-treated, and normal control mice on days 5, 10, and 15 (p<0.05). In addition, the lymphocyte levels in mice that received the extract dosages of 200 and 400mg/kg bw were non-significant compared with those of the normal and levamisole-treated mice on days 5, 10, and 15 (p>0.05; Figure 1).
On days 5, 10, and 15, the monocyte count in EHEC-infected control mice was substantially greater (p<0.05) than those in extract-treated, levamisole-treated, and normal control mice. Besides, the monocyte counts in the extract-treated (200 and 400mg/kg bw) and levamisole-treated mice were statistically similar on days 5, 10, and 15 (p>0.05). Further, on days 5, 10, and 15, the levels of monocytes in mice that received the extract doses of 200 and 400mg/kg bw were substantially higher relative to (p<0.05) levels of normal control mice (Figure 1).
The levels of eosinophils in EHEC-infected control mice were substantially higher than those in extract-treated, levamisole-treated, and normal control mice on days 10 and 15 (p<0.05). Besides, the eosinophils count in normal control mice were substantially lower than those of the other treatment groups on days 5 and 15 (p<0.05), although on day 10, the levels in the healthy control mice were nonsignificant with those of extract-treated and levamisole-treated mice (p>0.05; Figure 1).
The basophil levels were substantially greater in EHEC-infected control mice relative to those noted in other treatment groups on days 5, 10, and 15 (p<0.05). In addition, the basophil counts in extract-treated mice doses of 200 and 400mg/kg bw did not differ significantly and were comparable to levamisole-treated mice on days 5, 10, and 15 (p>0.05). Further, the basophil levels in normal control mice were substantially lower than the levels in other treatment groups on day 15 (p<0.05; Figure 1).
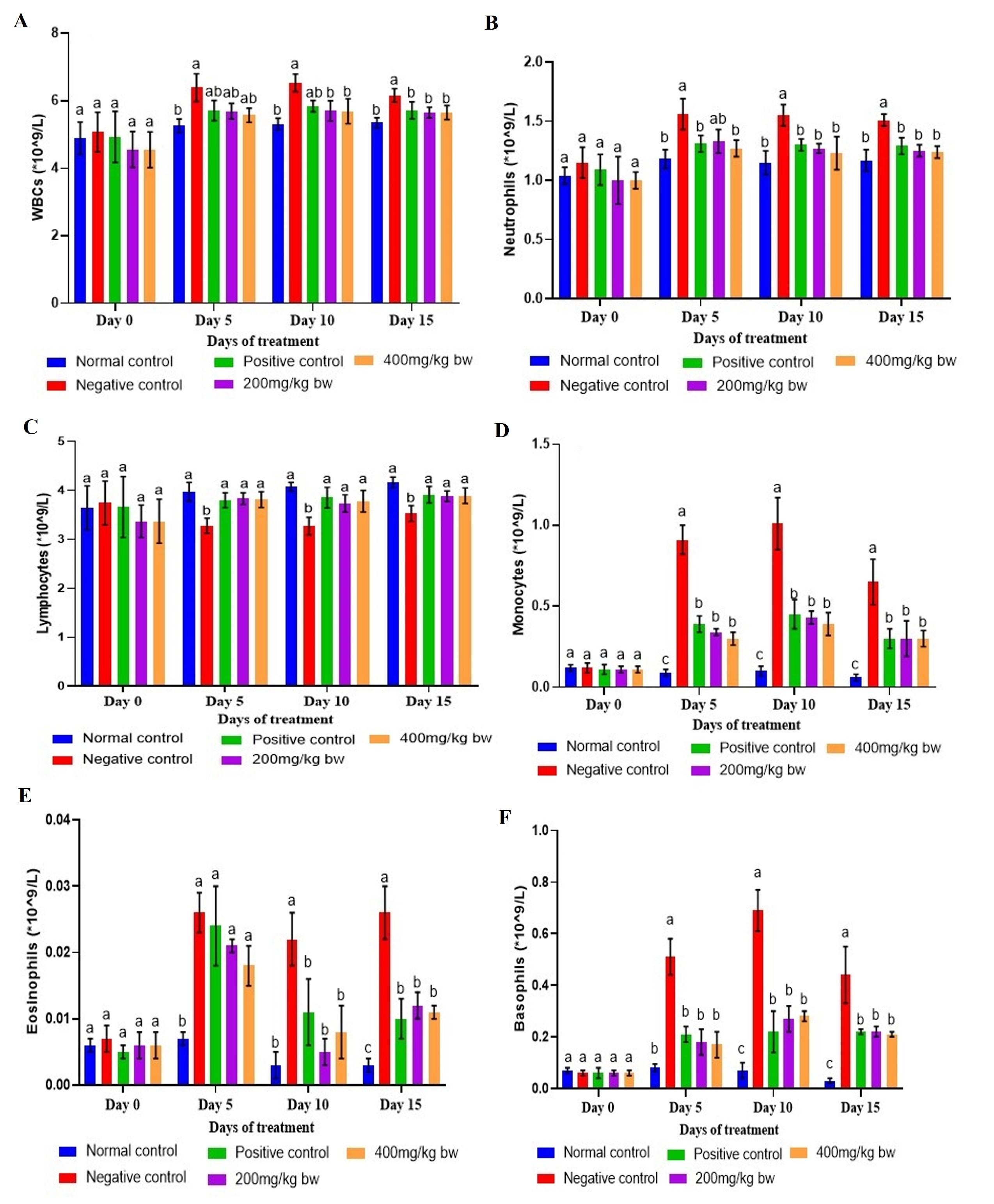
Effect of aqueous extract of T. striatus on levels of INF-γ in EHEC-infected mice
There was a substantial rise in the levels of INF-γ in EHEC-infected mice from day 7 onwards relative to those reported in the healthy control mice (p<0.05; Figure 2). On day 0, the INF-γ levels were insignificant in all the studied groups (p>0.05). Therapy with the extract, as well as levamisole significantly lowered the INF-γ levels compared to levels noted in the negative control mice from day 7 onwards (p<0.05). The INF-γ levels in mice that received the extract at the lower dose were significantly higher on days 7 and 14 of the study than those of mice that were administered the extract dose of 400mg/kg bw (p<0.05). On day 7, the statistical equivalence between levamisole and the extract dose of 200mg/kg bw was evident, extending to the two doses of the extract on days 14 and 21 (p>0.05; Figure 2).
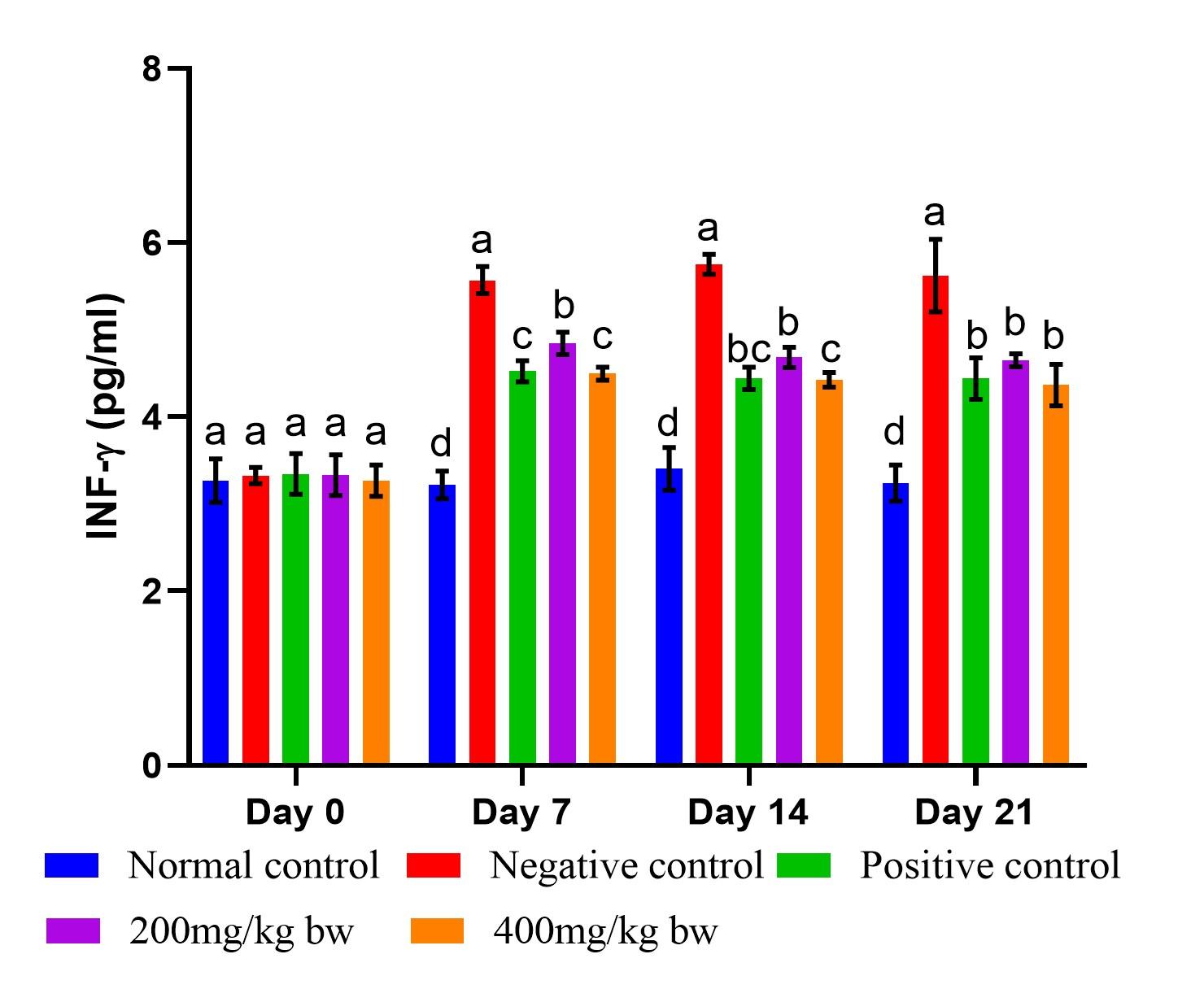
Effect of aqueous extract of T. striatus on levels of IL-4 in EHEC-infected mice
The mice that were infected with EHEC demonstrated a significant reduction in the IL-4 levels relative to the levels of mice in the healthy control mice (Figure 3). There was no significant variation in the IL-4 levels in all the studied groups on day 0 (p>0.05). The IL-4 levels in extract-treated mice at the two doses did not differ significantly in the entire study (p>0.05). The effect of levamisole on the IL-4 levels was nonsignificant relative to the levels noted in the extract-treated mice dose of 200mg/kg bw on days 14 and 21 (p>0.05). Starting from day 7, the normal control mice exhibited markedly elevated levels of IL-4 in comparison to those observed in the other examined groups (p<0.05). Conversely, the control mice infected with EHEC demonstrated significantly reduced levels of IL-4 on days 7, 14 and 21 of the experiment (p<0.05; Figure 3).
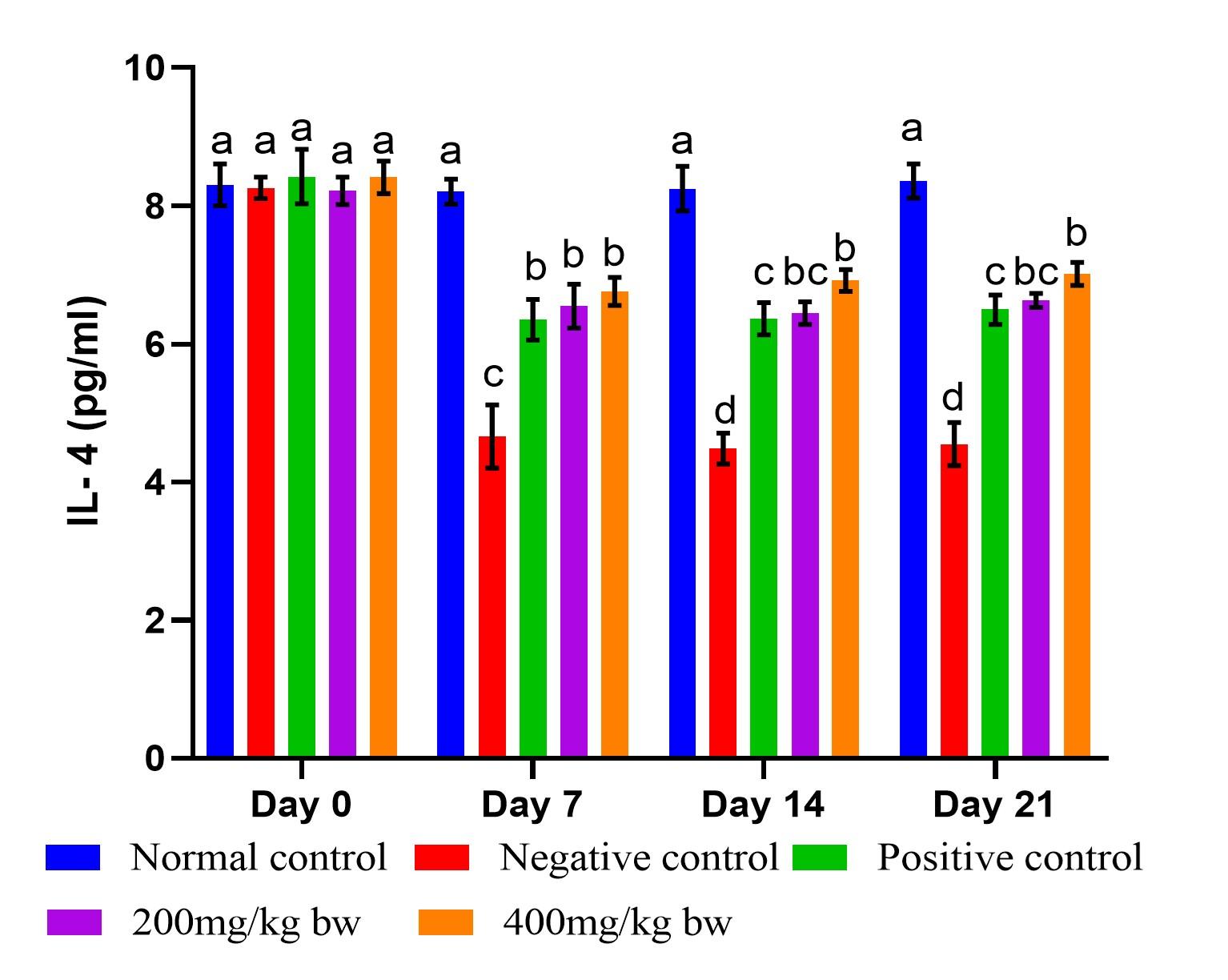
Effect of aqueous extract of T. striatus on levels of IgG in EHEC-infected mice
Infection of mice with EHEC caused a significant increase in IgG levels (p<0.05) from day 7 of the experiment (Figure 4). On day 7, the effect of the extract at the two doses did not differ significantly and statistically matched the effect of the reference drug (p>0.05). However, the effect of the extract dose of 400mg/kg bw had substantially higher (p<0.05) levels of IgG than the lower dose on days 14 and 21. The levels of IgG in mice treated with the reference drug showed no statistical significance difference when compared to mice that received the lower dose of the extract on days 14 and 21 (p>0.05) as illustrated in Figure 4.
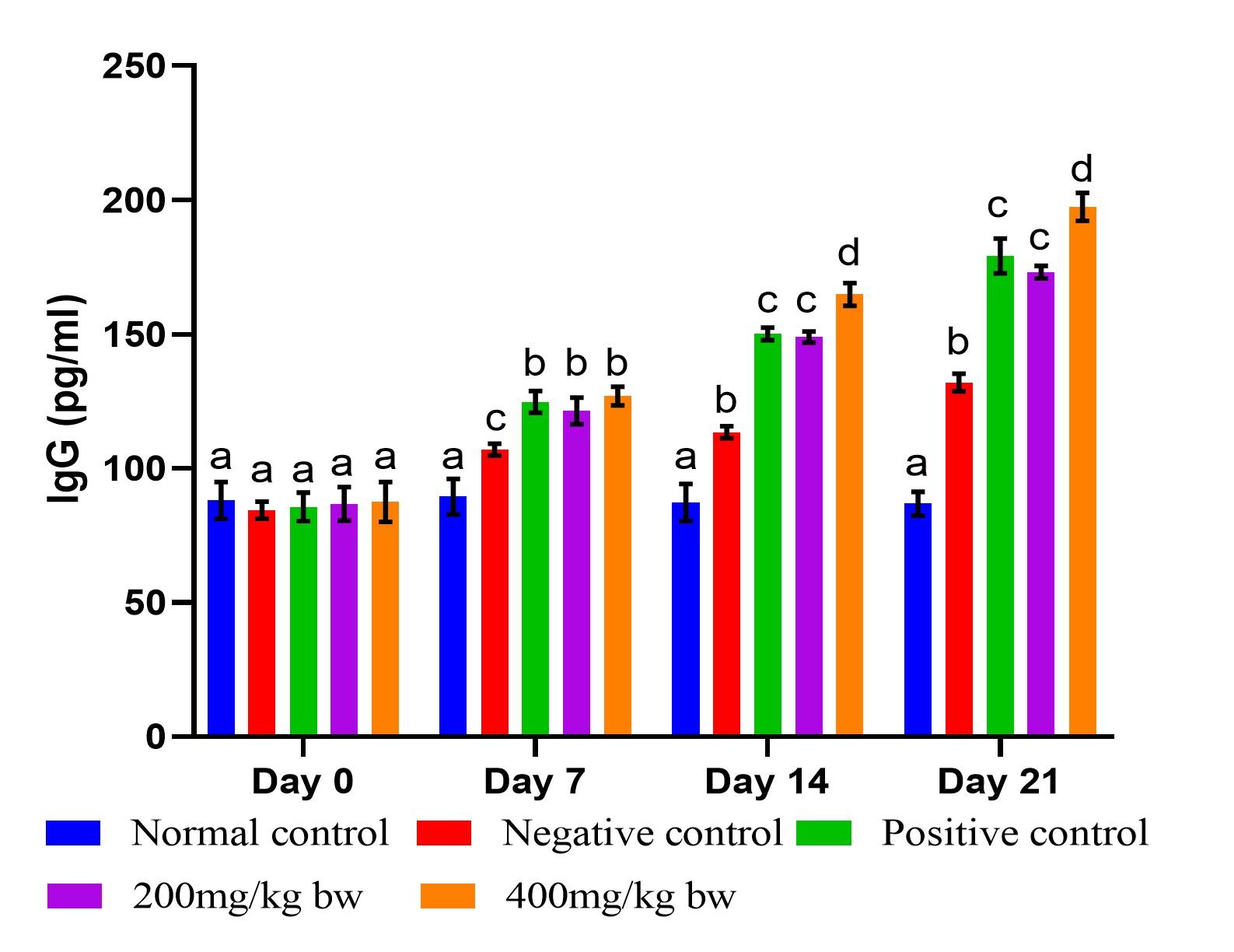
Qualitative phytochemical screening
Flavonoids, alkaloids, steroids, saponins, and phenols were qualitatively analyzed in T. striatus aqueous extract (Table 1).
Table 1. Phytochemical screening of T. striatus aqueous extract.
DISCUSSION
Comparatively, natural-origin drugs are safer and well-tolerated than synthetic drugs [27]. It has been reported that natural products are effective in treating a variety of illnesses, including immunosuppression [28]. This investigation aimed to evaluate the immunomodulatory effect of aqueous extract T. striatus in EHEC-infected mice, as well as qualitative phytochemical screening. The study demonstrated that the effect of T. striatus extract had an immunomodulatory effect following EHEC infection in mice. The extract also revealed the presence of phytocompounds that are associated with immunomodulatory effects. Phytocompounds have potent antioxidants, that affect various aspects of the immune system by adjusting the pro- and anti-oxidant equilibrium in favor of the antioxidant [29].
When a pathogen enters an organism, the innate immune system is the first to respond. It reacts quickly, but it lacks specificity and typically has a lesser effect compared to the adaptive immune response [3]. The innate immune system directly eliminates pathogens by discharging toxic chemicals (such as reactive oxygen species) from the phagocytes or by discharging toxic proteins by natural killer cells. The innate immune system also uses phagocytosis as a means of defense against invasive pathogens [30].
The effect of T. striatus extract doses of 200 and 400mg/kg bw showed a reduction in WBC, neutrophils, monocytes, eosinophils, and basophils levels, as well as an increase in the levels of lymphocytes in EHEC-infected mice, suggesting immunomodulatory effect (Figure 1). The EHEC produces Shiga toxin, which interacts with globotetraosylceramide (Gb3) on vascular endothelial cells resulting in suppression of protein synthesis, causing endothelial cell damage and cell death [31]. The lipopolysaccharides in the EHEC outer membrane also contribute to cytotoxic aggravation and damage of endothelial cells. These pathological alterations result in elevated WBC count [32].
In this study, it was noted that the levels of WBCs, neutrophils, monocytes, eosinophils, and basophils were significantly elevated after mice were infected with EHEC, indicating an inflammatory reaction. The mice that were administered aqueous extract of T. striatus restored the levels of WBCs, neutrophils, monocytes, eosinophils, and basophils close to those of the normal control mice. Phytochemicals including flavonoids, phenols, alkaloids, steroids, and saponins may be responsible for this effect. These phytocompounds have been reported to possess antioxidants and anti-inflammatory effects, an indicator of immunomodulatory activity [29]. In addition, the amelioration of WBC and differential counts could be attributed to the phagocytosis action of neutrophils, monocytes, eosinophils, and basophils against EHEC, thus eliminating the EHEC, as well as the need to produce these WBC differentials. Similar studies agreed with the findings of this study on the reduction of WBCs. A study by [32] reported that Fuzhuan brick-tea water extract ameliorated elevated levels of WBCs in mice infected with E. coli O157:H7.
The adaptive immune system can identify a pathogen uniquely and “recognize” it in the future. The two main types of lymphocytes involved in adaptive immunity are T cells and B cells [33]. T lymphocytes are crucial in antigen recognition and subsequent response of the immune system. T helper 1 (Th1) cells usually release IFN-γ and interleukin (IL)-2 that are crucial in cellular responses, while T helper 2 (Th2) cells secrete interleukins-(4, 5, and 13) that are linked with humoral immune and anti-parasitic responses [4,33]. B lymphocytes produce immunoglobulin (Ig) or antibodies that respond to a specific antigen. B cells can differentiate into short-lived plasma cells that synthesize immunoglobulins or can develop into long-lived plasma cells. Pathogen-specific immunoglobulins assist the immune system to recognize and eliminate them [3].
In this study, the levels of lymphocytes significantly reduced after mice were infected with EHEC, indicating an immune suppression [34]. However, the levels of the lymphocytes were significantly enhanced after infected mice were administered with the extract and the reference drug, suggesting an immunomodulatory effect. The bone marrow precursor cells give rise to lymphocytes, which are then selected, matured, and released in the thymus. Lymphocytes are classified as T cells and B cells [14]. B cells secrete immunoglobulins, which are antibodies, whereas T cells secrete chemicals (cytokines) that can either enhance or suppress inflammatory responses. Additionally, T-cell responses entail interacting with other cells to either destroy target cells or stimulate the production of antibodies. A distinctive characteristic of adaptive immunity is immunological memory, which is the growth of lymphocyte clones with a unique antigen-binding specificity so that a future contact elicits a stronger and faster response [14,33]. The increase in lymphocytes could be attributed to the bioactive constituents that were identified in this study.
In the current study, the levels of INF-γ significantly increased, while levels of IL-4 significantly declined in mice that were infected with EHEC, indicating an infection (Figures 2 and 3). Therapy with the extract doses of 200 and 400mg/kg bw, as well as reference drug, significantly increased levels of IL-4 and significantly lowered INF-γ levels, suggesting an immunomodulatory activity. Th1 and Th2 cells are mainly responsible for controlling cell-mediated and humoral immunity within an immune response. Th1 cells mostly generate IFN-γ and IL-2, which contribute to a cell-mediated response, while Th2 cells largely synthesize interleukins-(4, 5, and 10), which promote humoral response [35].
IFN-γ, a pro-inflammatory cytokine, is usually secreted by Th1 cells [35]. IFN-γ promotes immune response by enhancing differentiation of CD4+ T cells and phagocytosis of microbes, and activating neutrophils, macrophages, and natural killer cells [5]. IFN-γ also counteracts the suppressive effects of anti-inflammatory cytokines by inhibiting signaling pathways downstream events [36]. The mice that were administered T. striatus extract noted a decline in the levels of IFN-γ, suggesting an immunomodulatory effect. This could be attributed to the presence of phytocompounds that are associated with immunomodulatory effects such as flavonoids, phenols, alkaloids, and saponins. A study by [37] also reported a decline in the levels of IFN-γ after infected mice were administered Moringa oleifera and Marrubium vulgare extracts.
IL-4 is an anti-inflammatory cytokine that is secreted by Th2 cells, mast cells, basophils, and eosinophils [38]. It has a major role in humoral and adaptive immunity regulation. IL-4 stimulates B and T cells and has immunosuppressive effects, which may reduce levels of IFN-γ. Also, IL-4 reduces the production of Th1 cells, macrophages, and dendritic cells [39]. In this study, the levels of IL-4 significantly increased in EHEC-infected mice that received T. striatus extract at the two doses, indicating an immunomodulatory effect. A study by [35] also revealed a reduction in levels of IL-4.
Humoral immunity, particularly the production of antibodies, plays a crucial role in protecting against infections, including EHEC infection. The main types of antibodies generated by B cells include immunoglobulins A, D, E, G, and M [40]. Sufficient quantities of serum and secretory immunoglobulins, especially the antigen-specific antibodies IgG, are partly responsible for immunity against infection. The IgG exhibits targeted antibody activity against specific microbes or antigens. In the present study, the levels of IgG significantly increased after mice were infected with EHEC relative to normal control mice (Figure 4). The EHEC-infected mice that received T. striatus extract at the two doses noted a significant increase in the IgG levels compared to those of the negative control mice, suggesting an immunomodulatory activity. A study by [36] also reported similar findings.
CONCLUSION
This study concludes that aqueous extract of T. striatus exhibits immunomodulatory effects in mice infected with EHEC by improving the levels of WBCs and differential. In addition, the extract demonstrated a noteworthy decrease in the levels of INF-γ and increased levels of IL-4 and IgG in EHEC-infected mice. These findings indicate the potential use of the extract as an alternative immunomodulatory agent against EHEC infection.
ACKNOWLEDGMENTS
The authors acknowledge Elias Mendela, Pauline Otoli and Pamela Khasandi for providing laboratory technical support. The authors also wish to acknowledge the Institute of Primate Research where this research study was undertaken.
AUTHORS CONTRIBUTION
SWNC and WWR conceived the study idea and carried out laboratory work; WWR and OAL developed the study design. All authors participated in data analysis and interpretation, as well as the development of the manuscript.
CONFLICTS OF INTEREST
There is no conflict of interest among the authors.
References
- [1]Marshall JS, Warrington R, et al. An introduction to immunology and immunopathology. Allergy, Asthma & Clinical Immunology. 2018;14(2):1-0.
- [2]Delves PJ. Innate and adaptive systems of immunity. InThe Autoimmune Diseases 2020 (pp. 45-61). Academic Press.
- [3]Prabhu SR. An Introduction to Immunology and Immunopathology. InTextbook of General Pathology for Dental Students 2023 (pp. 73-80). Cham: Springer Nature Switzerland.
- [4]McDonald DR, Levy O. Innate immunity. InClinical immunology 2019 (pp. 39-53). Elsevier.
- [5]Widyaningsih TD, Martati ER, et al. Immunomodulatory effects of black cincau (Mesona Palustris BL.) supplement on Escherichia Coli strain O157-infected mice. Asian Journal of Pharmaceutical and Clinical Research. 2017;10(9):326.
- [6]Martinez-Medina M. pathogenic Escherichia coli: Infections and therapies. Antibiotics. 2021;10(2):112.
- [7]Gambushe SM, Zishiri OT, et al. Review of Escherichia Coli O157: H7 prevalence, pathogenicity, heavy metal and antimicrobial resistance, African perspective. Infection and Drug Resistance. 2022 :4645-73.
- [8]Fatima R, Aziz M. Enterohemorrhagic Escherichia Coli (EHEC). StatPearls [Internet]. 2020.
- [9]Wainwright CL, Teixeira MM, et al. Future directions for the discovery of natural product-derived immunomodulating drugs: An IUPHAR positional review. Pharmacological Research. 2022; 177:106076.
- [10]Nair A, Chattopadhyay D, et al. Plant-derived immunomodulators. InNew look to phytomedicine 2019 (pp. 435-499). Academic Press.
- [11]Kumar P, Rai S, et al. Classification, Mode of Action and Uses of Various Immunomodulators. InImmunomodulators and Human Health 2022 (pp. 3-38). Singapore: Springer Nature Singapore.
- [12]Yuandani, Jantan I, et al. Immunomodulatory effects and mechanisms of curcuma species and their bioactive compounds: A review. Frontiers in Pharmacology. 2021; 12:643119.
- [13]Singh MR, Yadav K, et al. Immune system and mechanism of immunomodulation. InPlants and Phytomolecules for Immunomodulation: Recent Trends and Advances 2022 (pp. 1-31). Singapore: Springer Nature Singapore.
- [14]Yang H, Choi K, et al. Immunoenhancing Effects of Euglena gracilis on a Cyclophosphamide-Induced Immunosuppressive Mouse Model. Journal of Microbiology and Biotechnology. 2022;32(2):228.
- [15]Zebeaman M, Tadesse MG, et al. Plants and Plant-Derived Molecules as Natural Immunomodulators. BioMed Research International. 2023; 2023.
- [16]Swaroop AK, Lalitha CM, et al. Plant Derived Immunomodulators; A Critical Review. Advanced Pharmaceutical Bulletin. 2022;12(4):712.
- [17]Pathak S, Fialho J, et al. Plant-based immunomodulators and their potential therapeutic actions. Journal of Exploratory Research in Pharmacology. 2022;7(4):243-56.
- [18]Paloi S, Kumla J, et al. Termite Mushrooms (Termitomyces), a Potential Source of Nutrients and Bioactive Compounds Exhibiting Human Health Benefits: A Review. Journal of Fungi. 2023; 9(1):112.
- [19]Nhi NT, KHANG DT, Dung TN. Termitomyces mushroom extracts and its biological activities. Food Science and Technology. 2022; 42:e125921.
- [20]Sitati CN, Ogila KO, et al. Phytochemical profile and antimicrobial activities of edible mushroom Termitomyces striatus. Evidence-Based Complementary and Alternative Medicine. 2021; 2021:1-0.
- [21]Savkovic SD, Villanueva J, et al. Mouse model of enteropathogenic Escherichia coli infection. Infection and Immunity. 2005;73(2):1161-70.
- [22]Farooq S, Shaheen G, et al. Preliminary phytochemical analysis: In-Vitro comparative evaluation of anti-arthritic and anti-inflammatory potential of some traditionally used medicinal plants. Dose-Response. 2022; 20(1):15593258211069720.
- [23]Anusmitha KM, Aruna M, et al. Phytochemical analysis, antioxidant, anti-inflammatory, anti-genotoxic, and anticancer activities of different Ocimum plant extracts prepared by ultrasound-assisted method. Physiological and Molecular Plant Pathology. 2022; 117:101746.
- [24]Mazzara E, Torresi J, et al. A Comprehensive Phytochemical Analysis of Terpenes, Polyphenols and Cannabinoids, and Micromorphological Characterization of 9 Commercial Varieties of Cannabis sativa L. Plants. 2022;11(7):891.
- [25]Hussen EM, Endalew SA. In vitro antioxidant and free-radical scavenging activities of polar leaf extracts of Vernonia amygdalina. BMC Complementary Medicine and Therapies. 2023; 2(1):1-2.
- [26]Qiu Z, Li CJ. Transformations of less-activated phenols and phenol derivatives via C–O cleavage. Chemical Reviews. 2020;120(18):10454-515.
- [27]Strzelec M, Detka J, et al. Immunomodulation—a general review of the current state-of-the-art and new therapeutic strategies for targeting the immune system. Frontiers in Immunology. 2023; 14:1127704.
- [28]Gurjar VK, Pal D. Natural compounds extracted from medicinal plants and their immunomodulatory activities. Bioactive Natural Products for Pharmaceutical Applications. 2021:197-261.
- [29]Maheshwari S, Kumar V, et al. Immunomodulatory potential of phytochemicals and other bioactive compounds of fruits: A review. Food Frontiers. 2022; 3(2):221-38.
- [30]Rusek P, Wala M, Druszczyńska M, Fol M. Infectious agents as stimuli of trained innate immunity. International Journal of Molecular Sciences. 2018; 19(2):456.
- [31]Newell DG, La Ragione RM. Enterohaemorrhagic and other Shiga toxin‐producing Escherichia coli (STEC): Where are we now regarding diagnostics and control strategies? Transboundary and emerging diseases. 2018; 65:49–71.
- [32]Wang Y, Xu A, Liu P, Li Z. Effects of Fuzhuan brick-tea water extract on mice infected with E. coli O157: H7. Nutrients. 2015; 7(7):5309–26.
- [33]Rich RR, Chaplin DD. The human immune response. In: Clinical Immunology. Elsevier; 2019. p. 3–17.
- [34]Kieckens E, Rybarczyk J, Li RW, Vanrompay D, Cox E. Potential immunosuppressive effects of Escherichia coli O157: H7 experimental infection on the bovine host. BMC genomics. 2016; 17:1-5.
- [35]Lin LH, Chi CH, Zhang XH, Chen YJ, Wang MF. Immunomodulatory Effects of Fruiting Body Extract and Solid-State-Cultivated Mycelia of Taiwanofungus camphoratus. Nutrients. 2019; 11(9):2256.
- [36]Song X, Yang Y, Li J, He M, Zou Y, Jia R, et al. Tannins extract from Galla Chinensis can protect mice from infection by Enterotoxigenic Escherichia coli O101. BMC Complementary Medicine and Therapies. 2021; 21(1):1–10.
- [37]Ajedi ASS, Widodo N, Widyarti S, Rifa’i M. Immunomodulatory effect of Moringa oleifera and Marrubium vulgare leaf aqueous extracts in BALB/c mice infected with Salmonella typhimurium. Drug Invention Today. 2019; 12(4).
- [38]Walker JA, McKenzie ANJ. TH2 cell development and function. Nature Reviews Immunology. 2018; 18(2):121–33.
- [39]Shaw DM, Merien F, Braakhuis A, Dulson D. T-cells and their cytokine production: The anti-inflammatory and immunosuppressive effects of strenuous exercise. Cytokine. 2018; 104:136–42.
- [40]Megha KB, Mohanan P V. Role of immunoglobulin and antibodies in disease management. International journal of biological macromolecules. 2021; 169:28–38.