Analysis of Staphylococcus epidermidis biofilm formation in several types of intravenous fluids based on time
Abstract
An intravenous catheter is a medical device used to inject intravenous fluid into the body. This procedure can cause coagulase-negative staphylococci (CoNS) bacteria such as Staphylococcus epidermidis penetrates the skin and forms biofilm on the catheter. Biofilms bring serious problems such as antibiotic resistance, the long-term effects that increase the length of staying in the hospital, cost, morbidity, and mortality. This research aimed to analyze the biofilm formation of S. epidermidis in several types of intravenous fluids based on time. This was a laboratory experimental research using the microtiter plate assay method and crystal violet coloring. Three 96-well microplates were inoculated with S. epidermidis in ringer lactate, 10% dextrose, 5% dextrose, normal saline, and gelafusal, where each plate was incubated at 3 different times of 24, 48, and 72 h, respectively. The results show that the optical density values of all intravenous fluids with bacteria within 24 and 48 h of incubation time did not show any significant differences compared to negative controls, while the 72 h treatments of 10% dextrose, 5% dextrose, and normal saline showed significant differences. This indicates that biofilms of S. epidermidis were not formed in intravenous fluids within 24 and 48 h of incubation time, however this bacterium started forming biofilm in 10% dextrose, 5% dextrose, and normal saline within 72 h of incubation time. In conclusion, the length of incubation time may influence biofilm formation.
INTRODUCTION
Nosocomial infection is a result of the interactions between pathogens, patients, practices, and places. Medical devices can be the source of infections [1], nowadays, the number of infections is associated with the higher application of medical devices and immunocompromised patients in the hospital [2]. Pathogenic bacteria can penetrate the skin through contamination during the application of a catheter [3] and cause bacteriemia [4, 5]. Generally, microorganisms causing nosocomial infections are biofilm producers [6]. The presence of biofilm poses the risk of decreasing susceptibility to antibiotics [7] and lead resistant infection to conventional drugs [8]. The long-term effects are often related to the length of hospitalization, economic burden, increased patient morbidity and mortality [9].
Coagulase-negative staphylococci (CoNS) is the most abundant constituent of the normal flora on the skin and mucous membranes of humans, but it can be opportunistic pathogen bacteria related to nosocomial infection [10]. The most common CoNS species causing the infection is S. epidermidis [11, 12]. Usually, these bacteria are single-celled or planktonic, but in some conditions, they can colonize to form biofilm, especially on moist and nutrient-rich surfaces [13, 14]. Staphylococcus can produce an extracellular polymer substances (EPS) matrix and form biofilm within 18-48 h of incubation in suitable condition [15].
An intravenous catheter is an important therapy for treating surgical patients and critical patients who have lost a lot of fluids such as dehydration due to vomiting, diarrhea, and shock [16], as parenteral nutrition in patients with certain medical conditions [17]. The insertion of an intravenous catheter must pay attention to the aspect of sterility so that microorganisms do not cause local infection in the puncture area and enter the blood vessels [18]. The maximum time for intravenous catheter application is 3 days, but if any signs of phlebitis such as redness, swelling, fever, and pain, the catheter must be removed immediately [19]. Crystalloid intravenous fluid is divided into 3 groups based on tonicity or electrolyte concentration i.e., isotonic such as ringer lactate, 5% dextrose, and normal saline (0.9% NaCl), hypertonic such as 10% dextrose, and hypotonic such as 0.45% NaCl [20]. While colloid intravenous fluid contains animal protein with a higher molecular weight than crystalloid [21].
A study demonstrated an increased prevalence of colonization and bacteremia on intravenous catheters at more than 48 h of installation [22]. However, the study about intravenous fluids and biofilm formation was very rare, the previous study was very old and only used one variable of time [23]. Thus, this study aimed to analyze the biofilm formation of S. epidermidis in several types of intravenous fluids such as ringer lactate (RL), 10% dextrose, 5% dextrose, normal saline (NS), and gelafusal based on incubation time of 24, 48, and 72 h.
MATERIALS AND METHODS
Ethical approval
The ethical approval of this research was issued by the Health Research Ethical Clearance Commission (KEPK), Faculty of Medicine, Universitas Airlangga with certificate number 149/EC/KEPK/FKUA/2022 on August 8th, 2022.
Bacterial strain culture and preparation of bacteria
S. epidermidis strain was obtained from the Microbiology Laboratory, Faculty of Medicine, Universitas Airlangga, Indonesia. S. epidermidis strain from the culture stock was grown on nutrient agar plate (NAP, Oxoid, Basingstoke, UK) and incubated in the incubator for about 24 h aerobically at 370C temperature. After verifying the purity of the strain, 3 to 4 identical morphologies were suspended in 5 mL of sterile distilled water [15]. The bacterial suspension was adjusted to match turbidity using 0.5 McFarland standard (1.5 x 108 CFU/mL). Then, the suspension was vortexed for at least 1 min and should be used at least 15 to 60 min after it was made.
Biofilm cultivation
The cultivation of biofilms was grown in a flatbottom microplate or microtiter plate 96-well (Biologix Europe GmbH, Germany). There were 3 microtiter plates prepared for different incubation times of 24, 48, and 72 h. Each well in the microtiter plate was filled with 180 µL of intravenous fluids and inoculated with 20 µL bacterial suspension. The intravenous fluid from the 1st column was RL (Otsuka, Indonesia), the second column was 10% dextrose (PT. Widatra Bhakti, Indonesia), the third column was 5% dextrose (Otsuka, Indonesia), the fourth column was NS (Otsuka, Indonesia), and the fifth column was gelafusal (Serumwerk Bernburg, Germany). The positive control consists of 200 µL trypticase soy broth (TSB, Oxoid, Basingstoke, Hampshire, England) only in the sixth column and the negative control consists of 200 µL RL, 10% dextrose, 5% dextrose, NS, gelafusal only in the seventh to eleventh column respectively. Each treatment in every column was repeated 6 times (rows A to F), so there were 66 filled wells. The microplates were covered with the cover, then incubated in an incubator (Heraeus) at 370C within 24, 48, and 72 h for the first, second, and third microplates, respectively.
Crystal violet staining
After incubation, the contents of the wells were decanted into a discarded container. Microplate was washed 3 times with 300 µL phosphate buffer saline (PBS, Laboratorium Bio Analitika, Surabaya, Indonesia) in each well using a micropipette with a gentle tap to remove planktonic bacterial cells that were not adherent to the microplate. Before staining, the microplate was drained in an inverted position. Then, 200 µL of 1% crystal violet dye (Laboratorium Bio Analitika, Surabaya, Indonesia) was added to each microplate well until it was submerged to stain the biofilm biomass in the microplate wells and left at room temperature for 20 min. The dye was removed, and the wells were washed again 3 times using PBS with the same method and then dried air in an inverted position. The last step before the reading of optical density was adding 200 µL of 96% ethanol (Laboratorium Bio Analitika, Surabaya, Indonesia) into each well and incubating for about 20 min.
Measurement of biofilm formation
The total biomass of biofilm after staining was measured by using a microplate reader (BIO-RAD iMarkTM) with 595 nm to get the OD value. The results obtained were interpreted by first looking for the cut-off value to distinguish biofilm-producing from non-biofilm-producing strains. There are 4 categories of grouping abilities to form biofilm [24], including OD < ODcut is non-biofilm-former (NBF), ODcut < OD < 2 ODcut is weak-biofilm-former (WBF), 2 ODcut < OD < 4 ODcut is moderate-biofilm-former (MBF), and OD > 4 ODcut is high-biofilm-former (HBF).
Statistical analysis
OD value was analyzed using Statistical Package for the Social Sciences (SPSS) 25.0 for Windows. Normality test with One Sample Kolmogorov-Smirnov Test and homogeneity test with Levene Test were used to find out the data distribution. The level of significance was determined using Kruskal-Wallis, then continue with the Mann-Whitney test and P < 0.05 was considered significant.
RESULTS
Based on the research, the average biofilm biomass (OD value) of S. epidermidis grown in intravenous fluids of RL, 10% dextrose, 5% dextrose, NS, and gelafusal within 24, 48, and 72 h of incubation time are presented in Table 1. The OD values of biofilm formation of S. epidermidis in TSB media as positive controls at every incubation time always increased, with the lowest value of 1.009+0.146 at 24 h and the highest value of 2.633+0.444 at 72 h. The OD values of biofilm of positive controls were significantly compared to inoculated intravenous fluids and negative controls (Figure 1-3).
Biofilm formation within 24 and 48 h of incubation times
All the inoculated intravenous fluids incubated for 24 and 48 h had similar OD values compared to negative controls (Table 1). Based on statistical test with Kruskal-Wallis, the OD values of biofilm formation within 24 and 48 h of incubation time were 0.002 and 0.008, respectively (P < 0.05), then continued with the Mann-Whitney test to determine the significance between treatments. The results of Mann-Whitney test on the formation of bacteria biofilm during incubation time of 24 and 48 h were not significant (Figures 1 and 2). The same letter in the graph indicated insignificant OD values between inoculated intravenous fluids and negative controls. The interpretations of biofilm formation showed that S. epidermidis grown in RL, 10% dextrose, 5% dextrose, NS, and gelafusal within 24 and 48 h of incubation time were NBF.
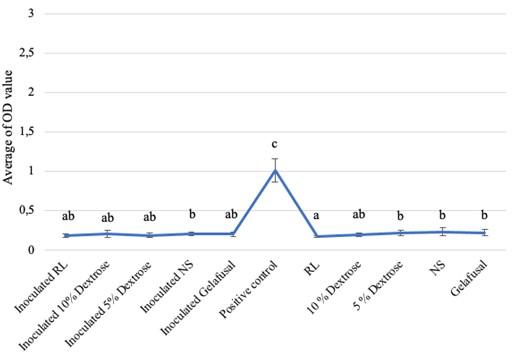
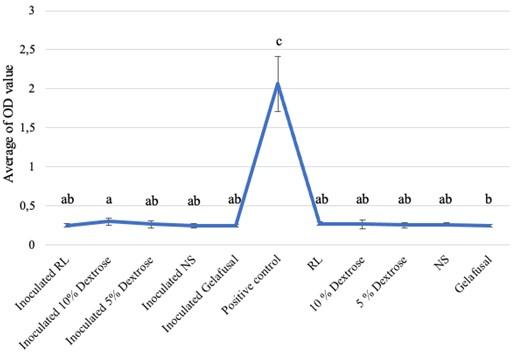
Table 1. Biofilm OD values obtained for S. epidermidis based on incubation time.
Biofilm formation within 72 h of incubation time
The average OD values of S. epidermidis biofilm formation within 72 h of incubation time grown in intravenous fluids had a slightly higher values than the negative controls (Table 1). The result of the Kruskal-Wallis test was 0.000 (P < 0.05), there were 3 treatments (10% dextrose, 5% dextrose, and NS) which had different significance compared to negative controls based on the Mann-Whitney test. The different letters in the graph indicated significant OD values between inoculated intravenous fluids of 10% dextrose, 5% dextrose, and NS compared to negative controls (Figure 3). However, the interpretation results of bacteria biofilm formation grown in RL, 10% dextrose, 5% dextrose, NS, and gelafusal were considered as NBF. This could be due to differences in standards between statistic and interpretation formulas.
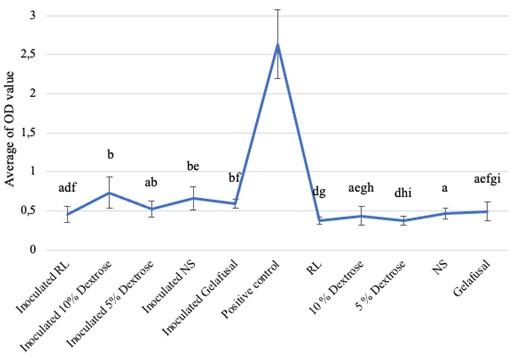
DISCUSSION
The data obtained from this study indicates that the length of incubation time results in different biofilm formations in intravenous fluids media. Statistically, in-vitro studies on biofilm formation tests with incubation times of 24 and 48 h did not show significant data, meanwhile at 72 h showed significant data. The biofilm biomass which is adherent on the base or wall of the microplate wells will bind to the crystal violet dye and this staining is performed to determine quantification through the absorbance of stained biofilms by measuring the OD value [25].
S. epidermidis used in this study were suspended in sterile distilled water, then inoculated into each intravenous fluid. Characteristics of sterile distilled water are pure, does not contain metals or anions, and has a pH of 7 (neutral) [26] which aims to provide a real condition where intravenous fluids given to patients are sterile. TSB is used as positive control media as it contains casein peptone which is rich in nitrogen, mineral, and vitamin, also soy peptone (papain) which is used as a dual-energy source for biofilm growth and formation [27].
In this research, biofilms were not formed in all intravenous fluids during 24 and 48 h of incubation time. In a previous study, the fluids passing through the catheter can affect microorganism growth but S. epidermidis did not grow well in intravenous fluids such as normal saline and dextrose solutions within 24 h of incubation time [23]. Incubation time is one of the factors influencing the growth of biofilms, time of biofilm formation is an important factor to see how quickly microorganisms can form biofilm in the right media [18].
Biofilm is defined as the association of microorganisms and EPS matrix which are sticky and slimy substances for binding bacteria to each other and attaching them to a material surface [13]. Some genes play role in S. epidermidis biofilm formation of the initial attachment stage including the PIA (polysaccharide intercellular adhesin) which functions to induce biofilm formation, the embp gene which functions in intercellular adhesion [28] and the Aap gene, protein related to accumulation gene which functions in primary attachment [29]. The genes involved in the maturation and dispersion stages are the dspB gene which functions as a PIA degrading enzyme [30] and the atlE gene which functions as an autolysin gene [28]. The metabolism of planktonic and biofilm cells of bacteria are different, tricarboxylic acid (TCA) or Krebs cycle is inactive in bacteria cells in the biofilm phase, either due to Krebs cycle inhibitors or gene mutations, which will increase the expression of the icaADBC operon in S. epidermidis [31]. A study proved that S. epidermidis isolates with icaA and/or icaD positively formed biofilm [32].
In this study, S. epidermidis cultivated in 10% dextrose, 5% dextrose, and normal saline started to form biofilms at 72 h of incubation time, although the values were slightly higher compared to negative controls. No previous report described the biofilm formation of S. epidermidis in intravenous fluids at 72 h of incubation time. However, a previous study showed that after 72 h of incubation, the morphology of S. epidermidis had changed, from planktonic phase to cell clusters which are defined as biofilm characteristics [33]. The clinical isolates from center venous catheters had identified the biofilm formation and 90% of the isolates were strong biofilm formers of S. epidermidis [34], so there is still a possibility that biofilm formed on catheter needles in patients’ body for three or more days can grow in intravenous fluids as media to continue forming biofilm and cause bacteremia.
Biofilm formation of S. epidermidis in 10% dextrose at 72 h incubation had the highest value, followed by normal saline, and 5% dextrose. The glucose molecule is higher in 10% dextrose than 5% dextrose, so there were more nutrients for bacteria to grow and form biofilm. Glucose is a carbon source used for the formation of biofilms [35]. S. epidermidis biofilms can be formed on media rich in carbon sources such as glucose, even maltose, lactose, and sucrose [36]. Research on biofilm formation with 0.5% glucose and 1.0% glucose addition to TSB media produces higher biofilms than biofilms grown on TSB media only [37]. Even the addition of 0.2% and 0.4% glucose can increase the bacteria biofilm biomass significantly [38]. Glucose metabolism produces short fatty acid chains which will decrease the pH of the medium [39] and suppress the agr (accessory gene regulator) quorum sensing system [40]. The acidic condition suppresses the production of extracellular protease [41], induces the association of biofilm matrix proteins on cell surfaces [42], and forms biofilm. Besides, the addition of 1.0% NaCl and 0.2% NaCl to TSB media also can increase the biofilm formation [37]. NaCl can activate the expression of the icaADBC operon [43]. The icaADBC operon is involved in the production of PIA, so biofilm-forming bacteria are able to induce biofilm formation [31].
CONCLUSION
In this study, we inoculated S. epidermidis in several types of intravenous fluids at three different times. The results showed that biofilm formation did not form at 24 and 48 h of incubation time in all intravenous fluids, whereas biofilms began to form at 72 h in some intravenous fluids. The OD values of all inoculated intravenous fluids were not statistically significant at 24 and 48 h of incubation time compared to negative controls. Within 72 h of incubation time, the OD values of inoculated 10% dextrose, 5% dextrose, and NS were statistically significant, while the inoculated RL and gelafusal were not statistically significant compared to negative controls. The length of incubation time supported by the rich-nutrient media may influence biofilm formation. The longer using of medical devices may cause the higher possibility of biofilm formation. Biofilm formation can be prevented by applying aseptically procedures, carrying out routine checks and maintenance. We have many shortcomings and limitations in this study, so further research can be carried out in the future.
ACKNOWLEDGEMENTS
We would like to say thank you to the Institute of Tropical Disease (ITD), Universitas Airlangga, Surabaya, Indonesia for agreeing to assist in measuring research data.
AUTHOR CONTRIBUTIONS
SFJ, ADWW, and RJS designed outlines and drafted the manuscript. SFJ performed the experiments, analyzed the data, and wrote the initial draft of the manuscript. ADWW and RJS reviewed the scientific contents described in the manuscript.
CONFLICTS OF INTEREST
There is no conflict of interest among the authors.
References
- [1]Jenkins DR. Prevention and control of infection, nosocomial infections and infection control. Medicine. 2021; 49(10): 638-642.
- [2]Davamani F, Jiun WW, Chitra E. Microbial biofilms and human associated with indwelling devices. In: Murthy PS, Sekar R, Thiyagarajan V, editors. Biofilm Control, Oxford: Alpha Science International Ltd; 2019.
- [3]Akhrass FA, Hachem R, Mohamed JA, Tarrand J, Kontoyiannis DP, Chandra J, et al. Central venous catheter-associated nocardia bacteremia in cancer patients. Emerg Infect Dis. 2011; 17(9): 1651-1658.
- [4]Cillespie S, Bamford K. Medical Microbiology and Infection at A Glance, 4th ed. John Wiley and Sons, Ltd: West Sussex, 2012.
- [5]Bresco MS, Harris LG, Thompson K, Stanic B, Morgenstern M, O'Mahony L, et al. Pathogenic mechanisms and host interactions in Staphylococcus epidermidis device-related infection. Front Microbiol. 2017; 8.
- [6]Cangui-Panchi SP, Nacato-Toapanta AL, Enriquez-Martinez LJ, Reyes J, Garzon-Chavez D, Machado A. Biofilm-forming microorganisms causing hospital-acquired intravenous catheter: a systematic review. Current Research in Microbial Sciences. 2022; 3.
- [7]Rivani E, Arfijanto MV, Widodo ADW, Vancomycin for methicillin-resistant Staphylococcus aureus biofilm eradication is associated with the emergence of heterogeneous vancomycin intermediate Staphylococcus aureus. International Journal of Health Science. 2022; 6(S9): 811-818.
- [8]Galdiero E, Ricciardelli A, D’Angelo C, de Alteriis E, Maione A, Albarano L, et al. Pentadecanoic acid against Candida albicans-Klebsiella pneumonia biofilm: towards the development of an anti-biofilm coating to prevent polymicrobial infections. Research in Microbiology. 2021; 172.
- [9]Kart D, Tavernier S, Acker HV, Nelis HJ, Coenye T. Activity of disinfectants against multispecies biofilms formed by Staphylococcus aureus, Candida albicans and Pseudomonas aeruginosa. Biofouling. 2014; 30(3): 377-383.
- [10]Piette A, Verschragen G. Role of coagulase-negative staphylococci in human disease. Veterinary Microbiology. 2009; 134: 45-54.
- [11]Gkenzi D, Kolyva S, Spiliopoulou I, Marangos M, Dimitriou G. Treatment options for persistent coagulase negative staphylococcal bacteremia in neonates, Curr Pediatr Rev. 2016; 12(3): 199-208.
- [12]Riedel S, Morse SA, Mietzner T, Miller S. Jawetz, Melnick, & Adelberg’s Medical Microbiology, 28th ed. McGraw-Hill: New York, 2019.
- [13]Kanematsu H, Barry DM. Formation and control of biofilm in various environments. Springer: Singapore, 2020.
- [14]Roy PK, Song MG, and Park SY. The inhibitory effect of quercetin on biofilm formation of Listeria monocytogenes mixed culture and repression of virulence. Antioxidants. 2022; 11(9): 1733.
- [15]Stepanovic S, Vukovic D, Hola V, Bonaventura GD, Djukic S, Cirkovic I, et al. Quantification of biofilm in microtiter plates: overview of testing conditions and practical recommendations for assessment of biofilm production by staphylococci. APMIS. 2007; 115(8): 891-899.
- [16]Stoelting RK, Rathmell JP, Flood P, Shafer S. Handbook of Pharmacology and Physiology in Anesthetic Practice, 3rd ed. Wolters Kluwer Health: Philadelphia, 2015.
- [17]Braga M, Ljungqvist O, Soeters P. ESPEN guidelines on parenteral nutrition: surgery. Clin Nutr. 2009; 28(4): 378.
- [18]Ariningrum D, Subandono J. Buku Pedoman Keterampilan Klinis Pemasangan Infus untuk Semester 7. Universitas Sebelas Maret Surakarta: Solo, Indonesia, 2018.
- [19]Mulyadi Y. Standar Prosedur Operasional Pemasangan Infus. RSUD Dr Soetomo: Surabaya, Indonesia, 2017.
- [20]Butterworth JF, Mackey DC, Wasnick JD. Morgan dan Mikhail’s Clinical Anesthesiology, 5th ed. Mc-Graw Hill: New York, 2013.
- [21]Gray A, Wright J, Goodey V, Bruce L. Injectable Drugs Guide. Pharmaceutical Press: London, 2011.
- [22]Soleimani G, Zahedani SS, Mousavi SHS, Soleimani A, Parsa M, Bigzadi VV, et al. Colonization and Bacteremia due to Peripheral Venous Catheters in Hospitalized Children, Biomed J Sci & Tech Res. 2021; 34(1): 26408-26412.
- [23]Garcia-Caballero J, Herruzo-Cabrera H, Vera-Cortes ML, de Lorenzo AGD, Vazquez-Encinar A, Garcia-Caballero, F, et al. The growth of micro-organisms in intravenous fluids. Journal of Hospital Infection. 1985; 6(2): 154–157.
- [24]Singh AK, Prakash P, Achra A, Singh GP, Das A, Singh RK. Standardization and classification of in vitro biofilm formation by clinical isolates of Staphylococcus aureus. J Glob Infect Dis. 2017; 9(3): 93-101.
- [25]Nuryastuti T. Current In Vitro Assay to Determine Bacterial Biofilm Formation of Clinical Isolates, J Med Sci. 2014; 46(3): 142-152. doi:10.19106/JMedScie004603201406.
- [26]Indrawati T, Ningsih NID. Penerapan statistika proses control dalam pengamatan sifat fisika dan kimia air buangan dari air conditioning (AC). Integrated Lab Jurnal. 2018; 6(2): 85-92.
- [27]Wahyudi D, Soetarto ES. Pembentukan Biofilm Pseudomonas aeruginosa pada beberapa media cair. Journal of Pharmacy. 2021; 10(2): 35-40.
- [28]Büttner H, Mack D, Rohde H. Structural basis of Staphylococcus epidermidis biofilm formation: mechanisms and molecular interactions. Frontiers in Cellular and Infection Microbiology. 2015; 5(14): 1-15
- [29]Schaeffer CR, Woods KM, Longo GM, Kiedrowski MR, Paharik AE, Buttner H, Christer M, Boissy RJ, Horswill AR, Rohde H, Fey PD. Accumulation-associated protein enhances Staphylococcus epidermidis biofilm formation under dynamic conditions and is required for infection in a rat catheter model. Infection and Immunity. 2015; 83(1): 214-226.
- [30]Tan Y, Ma S, Liu C, Yu W, Han F. Enhancing the stability and antibiofilm activity of DspB by immobilization on carboxymethyl chitosan nanoparticles. Microbiological Research. 2015; 178: 35-41
- [31]Martinez-Garcia S, Peralta H, Betanzos-Cabrera G, Chavez-Galan L, Rodriguez-Martinez S, Cancino-Diaz ME, et al. Proteomic comparison of biofilm vs. planktonic Staphylococcus epidermidis cells suggests key metabolic differences between these conditions. Research in Microbiology. 2021; 172.
- [32]Rachmawati D, Kuntaman, Alimsardjono L. The correlation between icaA and icaD genes with biofilm formation Staphylococcus epidermidis in vitro. Fol Med Indones. 2019; 55(4): 251-259.
- [33]Bottagisio M, Soggiu A, Piras C, Bidossi A, Greco V, Pieroni L, et al. Proteomic analysis reveals a biofilm-like behavior of planktonic aggregates of Staphylococcus epidermidis grown under environmental pressure/stress. Frontier in Microbiology. 2019; 10.
- [34]Cherifi S, Byl B, Deplano A, Nagant C, Nonhoff C, Denis O, et al. Genetic characteristics and antimicrobial resistance of Staphylococcus epidermidis isolates from patients with catheter-related bloodstream infections and from colonized healthcare workers in a Belgian hospital. Annals of Clinical Microbiology and Antimicrobials. 2014; 13(20).
- [35]Uribe-Alvarez C, Chiquete-Felix N, Contreras-Zentella M, Guerrero-Castillo S, Pena A, Uribe-Carvajal S. Staphylococcus epidermidis: metabolic adaptation and biofilm formation in response to different oxygen concentrations. FEMS Pathogens and Disease. 2016; 74(1). doi:10.1093/femspd/ftv111.
- [36]Zou M, Liu D. Effects of carbon sources and temperature on the formation and structural characteristics of food-related Staphylococcus epidermidis biofilms. Food Science and Human Wellness. 2020; 9(4): 370-376.
- [37]Lade H, Park JH, Chung SH, Kim IH, Kim JM, Joo HS, et al. Biofilm formation by Staphylococcus aureus clinical isolates is differentially affected by glucose and sodium chloride supplemented culture media. J Clin Med. 2019; 8(11).
- [38]Lindarto WW, Wasito EB, Debora K. Effect of glucose induction on biofilm density in clinical isolate Acinetobacter baumannii patients in intensive care unit of Dr. Soetomo hospital Surabaya. Fol Med Indones. 2020; 56(2): 118-126.
- [39]Olson ME, King JM, Yahr TL, Horswill AR. Sialic acid catabolism in Staphylococcus aureus. J Bacteriol. 2013; 195(8): 1779–1788.
- [40]Regassa LB, Novick RP, Betley MJ. Glucose and nonmaintained pH decrease expression of the accessory gene regulator (agr) in Staphylococcus aureus. Infect Immun. 1992; 60(8): 3381–3388.
- [41]Boles BR, Horswill AR. Staphylococcal biofilm disassembly. Trends in Microbiology, 2011; 19(9): 449–455.
- [42]Foulston L, Elsholz AKW, DeFrancesco AS, Losick R. The extracellular matrix of Staphylococcus aureus biofilms comprises cytoplasmic proteins that associate with the cell surface in response to decreasing pH. MBio. 2014; 5(5).
- [43]O’Neill E, Pozzi C, Houston P, Smyth D, Humphreys H, Robinson DA, et al. Association between methicillin susceptibility and biofilm regulation in Staphylococcus aureus isolates from device-related infections. J Clin Microbiol. 2007; 45(5): 1379–1388