Development of gelatin particles encapsulating PDGF-BB aptamer for the efficient drug delivery system
Abstract
Drug delivery systems (DDSs) improve therapy through controlled release and targeted delivery, utilizing recognition elements, therapeutic agents, and carriers. Among various recognition elements, aptamers have gained attention for their high affinity and specificity. Gelatin, a biocompatible and biodegradable material, shows potential for DDSs due to its favorable properties. This study investigated gelatin-based particles for aptamer delivery, focusing on their preparation, characterization, and evaluation. Gelatin particles loaded with platelet-derived growth factor-BB (PDGF-BB) aptamer were prepared using glutaraldehyde as a crosslinker, with sodium dodecyl sulfonate (SDS) and Tween 20 as stabilizers. The particles were characterized through spectroscopy techniques, dynamic light scattering (DLS), and scanning electron microscopy (SEM). The reaction between aldehyde and amine groups was confirmed, and the effect of surfactants on particle formation was examined. Aptamer release and stability were also studied. The ninhydrin assay and Fourier-transform infrared (FTIR) analysis confirmed successful crosslinking between gelatin and glutaraldehyde, forming stable particles. Zeta potential measurements showed that both SGP and TGP maintained stability within the range of -30 to -60 mV. Particle size analysis via DLS and SEM revealed that increasing surfactant concentration led to larger particles, with sizes ranging from 95 to 120 nm. Weight loss studies demonstrated that SGP followed zero-order kinetics while TGP exhibited first-order degradation, with both retaining over 90% of their weight within 72 hours. Aptamer release studies showed that SGP had a higher release rate than TGP, correlating with their degradation profiles. Finally, electrophoresis confirmed the integrity of released aptamers, emphasizing the protective role of gelatin particles. Overall, gelatin-based particles show promise for aptamer delivery, offering stability and controlled release. These findings contribute to the advancement of effective DDSs for targeted therapeutic delivery.
INTRODUCTION
Drug delivery systems (DDSs) have been applied in various therapeutic drugs because they can be modified to control the drug release rate and deliver drugs to target areas or organs in the body [1]. In addition, the use of DDSs offers several advantages over traditional free drugs, including improvement of water solubility, enhancement of cellular penetration, and controllability of pharmacokinetics and body distribution [1]. DDSs commonly consist of recognition elements, therapeutic components, and drug-carrier materials [2]. The recognition ability of DDSs comes from binding ligands that have been attached to the systems. Some reported binding ligands include monoclonal antibodies [3], transferrin [4], various peptides [5], folate [6], and aptamers [7]. The therapeutic components are active ingredients used for specific treatments such as cancers [8], disorders [9], and diseases [10]. The active ingredients could be small drug molecules (e.g., anti-cancer drugs) or bio-macromolecules (e.g., proteins and oligonucleotides) [11]. The drug-carrier materials should exhibit biocompatible and biodegradable properties with less toxicity [12]. In this regard, natural-based biomaterials such as gelatin [13], chitosan [14], and alginate [15] are common and attractive candidates for developing DDSs. Though DDSs have promising advantages, there are a few drawbacks to oligonucleotide delivery, such as limited absorption, loading efficiency, and release kinetics [16]. Besides, natural-based biomaterials might not be feasible to use due to their limitations on enzymatic degradation or physical encapsulation [17]. Therefore, this work aims to seek a better understanding of DDSs in a platform of gelatin-based particles for the delivery of aptamers, a binding functional oligonucleotide that could enhance targeted therapeutic delivery efficacy.
Gelatin is a polypeptide-based biological material derived from collagen [18]. It exhibits nonimmunogenic, biocompatible, and biodegradable properties [18]. Gelatin is used in various applications, including the food industry, pharmaceutical drugs, and medical materials [19]. Many researches have focused on further improving gelatin properties by modifying its functional groups with chemical reactions [20]. In addition, gelatin has been prepared as nano/micro particles applied for the delivery system for a number of molecules such as growth factors and plasmid DNA [21, 22]. This inspires us that gelatin is promising material for the delivery of aptamers, nucleic acid-based macromolecules.
Aptamers are single-stranded DNA (ssDNA) or RNA sequences that fold into secondary and three-dimensional conformation and inherit recognition ability with high affinity and specificity [23]. Targets for aptamer binding include proteins, small molecules, metal ions, bacterial cells, viruses, cancer cells, and even live cells [24]. Aptamers also have many features, including small size, easy modification, low immunogenicity, and high selectivity [25]. These properties make aptamers good candidates for replacing antibodies. The aptamers’ nano-drug delivery systems have been modified and developed in recent years to improve the therapeutic efficacy and decrease unwanted side effects. Zhan et al. constructed a nanomedicine in a platform of a DNA tetrahedron composed of AS1411 aptamer and 5-fluorouracil (5-FU), an antimetabolite drug [26]. Their results showed that this nanomedicine could exhibit high therapeutic efficacy on target cells when compared to free 5-FU. The effective recognition ability of aptamers to various proteins on the cell membranes or in the blood circulation modulators makes aptamers promising therapeutic agents [27].
This research aimed to prepare PDGF-BB aptamer-loaded gelatin particles by using glutaraldehyde as a cross-linker and sodium dodecyl sulfonate (SDS) and tween 20 as particle stabilizers. The particles were characterized by spectroscopy techniques, light scattering, and scanning electron microscopy. The reaction between aldehyde and amine groups was confirmed by ninhydrin assay and FTIR spectroscopy. The effect of surfactant on particle formation was investigated. The PDGF-BB aptamer was incorporated into the prepared particles. The aptamer released from the particles and its stability were studied.
MATERIALS AND METHODS
Reagents
Acetic acid, analytical-grade ethanol, gelatin from bovine skin, glutaraldehyde, potassium bromide (KBr), and ninhydrin reagent were purchased from Sigma-Aldrich (Saint Louis, Missouri). Glycine and SDS were purchased from Bio-Rad laboratories (Hercules, California). Phosphate-buffered saline (PBS) and Tween 20 were obtained from Fisher Scientific (Rockford, Illinois). The PDGF aptamer was sourced from Integrated DNA Technologies (Asia Pacific Production, Singapore), and the sequence is 5’-ACA GGC TAC GGC ACG TAG AGC ATC ACC ATG ATC CTG-3’.
Preparation of gelatin particle
Gelatin was dissolved in deionized (DI) water at a concentration of 40% w/v. The resulting solution was then diluted to 2% w/v using surfactant solutions (SDS and Tween 20) with surfactant-to-critical micelle concentration (CMC) ratios of 0.4, 2.0, and 10.0. CMC of SDS and Tween 20 reported in the product description was 8 and 0.02 mM, respectively. Subsequently, 20 mL of the gelatin-surfactant solution was mixed with 100 mL of ethanol using a hot plate stirrer (SCILOGEX, Rocky Hill, Connecticut), stirred at 1,500 rpm, 60°C for 15 minutes, and further combined with 150 µL glutaraldehyde for crosslinking. The reaction proceeded for 2 hours, after which cross-linked gelatin particles were separated via centrifugation at 3000 rpm (GYROZEN Mini centrifuge, Seoul, South Korea) for 20 minutes and freeze-dried overnight (Christ Alpha 2-4 LSCplus, Osterode am Harz, Germany). The gelatin particles prepared in the presence of SDS and Tween 20 were named SGP and TGP, respectively. Here, SGP and TGP represent particles synthesized in the presence of SDS and Tween 20, respectively.
Determination of amine group in gelatin particle by ninhydrin assay
Unmodified gelatin and gelatin particles were suspended in 0.05% (v/v) acetic acid at a concentration of 0.01% (w/v). These suspensions (2 mL) were mixed with 1 mL of 2% (w/v) ninhydrin solution and heated in a boiling water bath for 10 minutes. After cooling to room temperature, the mixtures were transferred to mL of 95% ethanol, and absorbance was recorded at 570 nm using a UV-Vis spectrophotometer (PharmaSpec, Shimadzu, Japan).
Fourier transform infrared spectrometer
Unmodified gelatin and gelatin particles, mixed with KBr and pressed into discs, were analyzed using a Fourier transform infrared (FTIR) spectrometer (Perkin Elmer, Spectrum GX) to characterize the functional groups of the gelatin particles.
Dynamic light scattering and Zeta potential measurement
The size distribution and zeta potential of gelatin particles were measured using a Nano Particle Analyzer (HORIBA, Nano Partica SZ-100) at 25 °C with a scattering angle of 173°. The particles were suspended in DI water at a concentration of 0.02 mg/mL for measurements.
Scanning electron microscopy
Dried particles adhered to an adhesive scanning electron microscopy (SEM) stub and coated with gold were examined for particle morphology using SEM (JSM 5410, JEOL) at 40000x magnification, 5kV. The size of gelatin particles was manually measured from the SEM images.
Examination of weight loss
0.02 g of gelatin particles were placed in microcentrifuge tubes containing 500 µL of PBS and incubated at 25°C. During the incubation period, samples were shaken by an orbital shaker (N-Biotek, South Korea). At predetermined times, the particle suspension was centrifuged at 3,000 rpm. The particles were removed from the PBS, dried in a vacuum chamber overnight using a single-stage pump (SR Brand, SPG-1A model, Bangkok), and weighed (TC205, Denver Instrument Company, Denver, Colorado) to determine the weight loss. The percentage weight loss was calculated from the ratio between the weight of dried particles and the initial weight. The experiment was conducted in triplicate.
Preparation of aptamer loading gelatin particle
Gelatin particles (0.50g) were incubated in 5 mL of 5 µM aptamer under agitating conditions overnight. The suspension was centrifuged at 3,000 rpm, and a supernatant (4.5 mL) was transferred to an additional test tube, with 4.5 mL fresh PBS refilled. The amount of PDGF-BB aptamer in the supernatant was determined by absorbance at 260 nm using a Nanodrop spectrophotometer (BecThai, Thailand). Aptamer loading was calculated by the following equation, , where is an initial amount of PDGF-BB aptamer (mg), is an amount of the aptamer remained in supernatant after particle incubation [28].
Aptamer release
Aptamer-loaded gelatin particles were incubated in 5 mL of PBS as a release medium. This particle suspension was kept in a 15 mL capped tube and continuously agitated with the orbital shaker at 25 °C. At predetermined time points, 100 µL of release medium was collected, and fresh PBS was refilled. Aptamer concentration in the release medium was quantified by the spectrophotometer. The aptamer cumulative release was calculated using the ratio between the amount of aptamer detected at the predetermined time and the total amount of aptamer released. All experiments were performed in triplicate [29].
Stability of released aptamer
To confirm the stability of released aptamers, the aptamer solution collected at each specified time during the release study was subjected to 10% polyacrylamide gel electrophoresis, and the gel was imaged using Gel documentation system (GelDoc Go, Bio-Rad Laboratories).
Statistical analysis
All data are presented as the mean ± standard deviation (SD) from at least three replicate samples. Comparisons between the control and study groups were conducted using Student’s t-test, with statistical significance defined as P < 0.05.
RESULTS
Role of surfactant on crosslink reaction
According to the ninhydrin assay results (Figure 1), the unmodified gelatin (UG) exhibited a higher count of amine groups compared to gelatin particles (e.g., SGP and TGP). This observation suggests a crosslink reaction involving amino and aldehyde groups, resulting in the formation of solid gelatin particles. While surfactant concentrations were altered, the quantity of glutaraldehyde remained constant across all preparation formulations. As a result, the remaining amine groups detected after the reaction in all formulations were consistent in terms of magnitude. The crosslink reaction was further validated through FTIR analysis (Figure 2). For TGP and SGP, a distinct peak between 1640-1690 cm⁻¹ was identified, corresponding to C=N stretching. Notably, this peak was absent in UG. The C=N stretching signifies the presence of an aldimine bond formed by the reaction between amino groups from gelatin and aldehyde groups from glutaraldehyde. Additionally, gelatin displayed amide peaks around 1652 cm⁻¹, 1539 cm⁻¹, and 1241 cm−1[30]. A marginal shift in these peaks observed in TGP and SGP serves as another indication of the crosslink reaction [31].
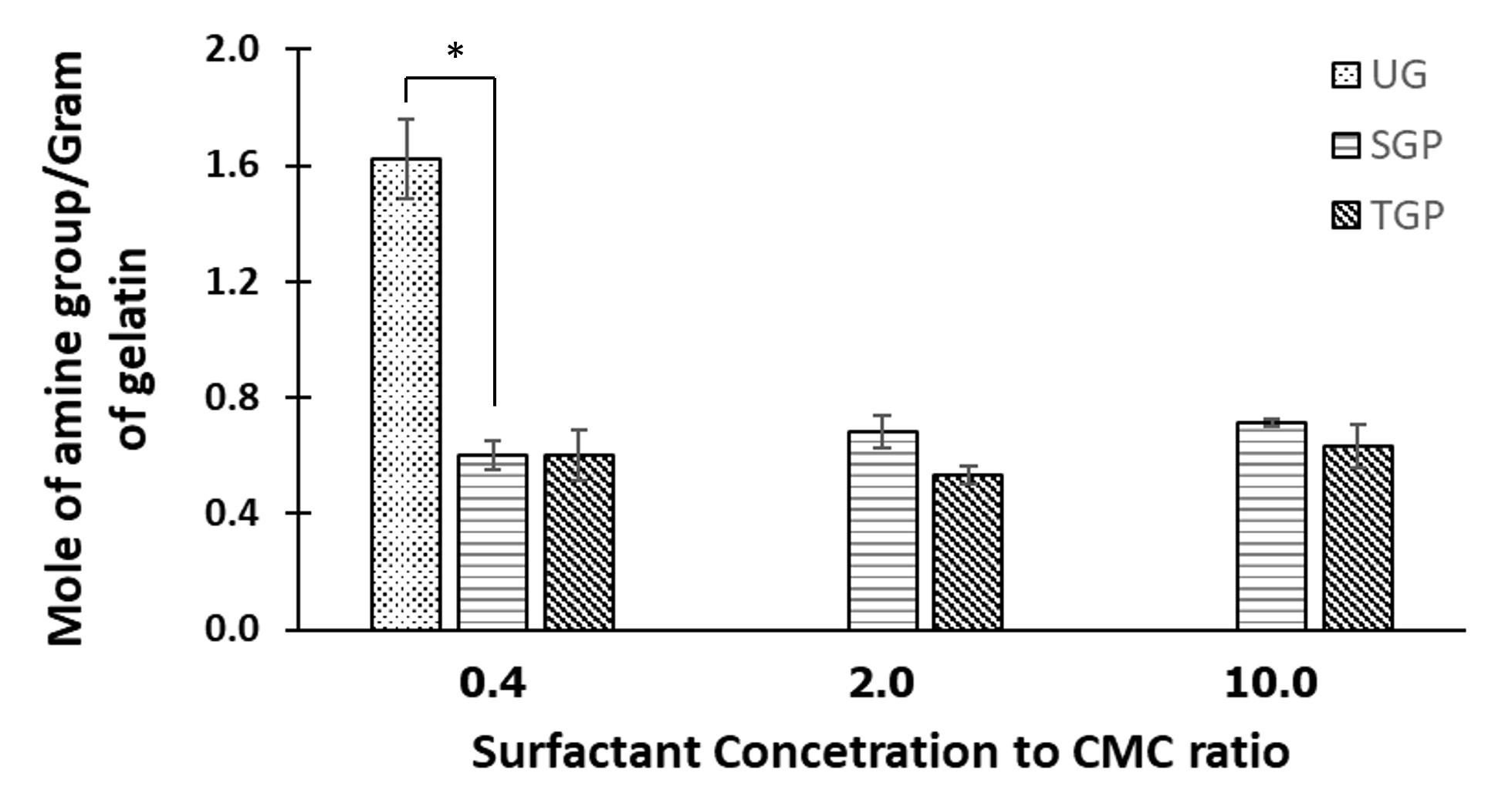
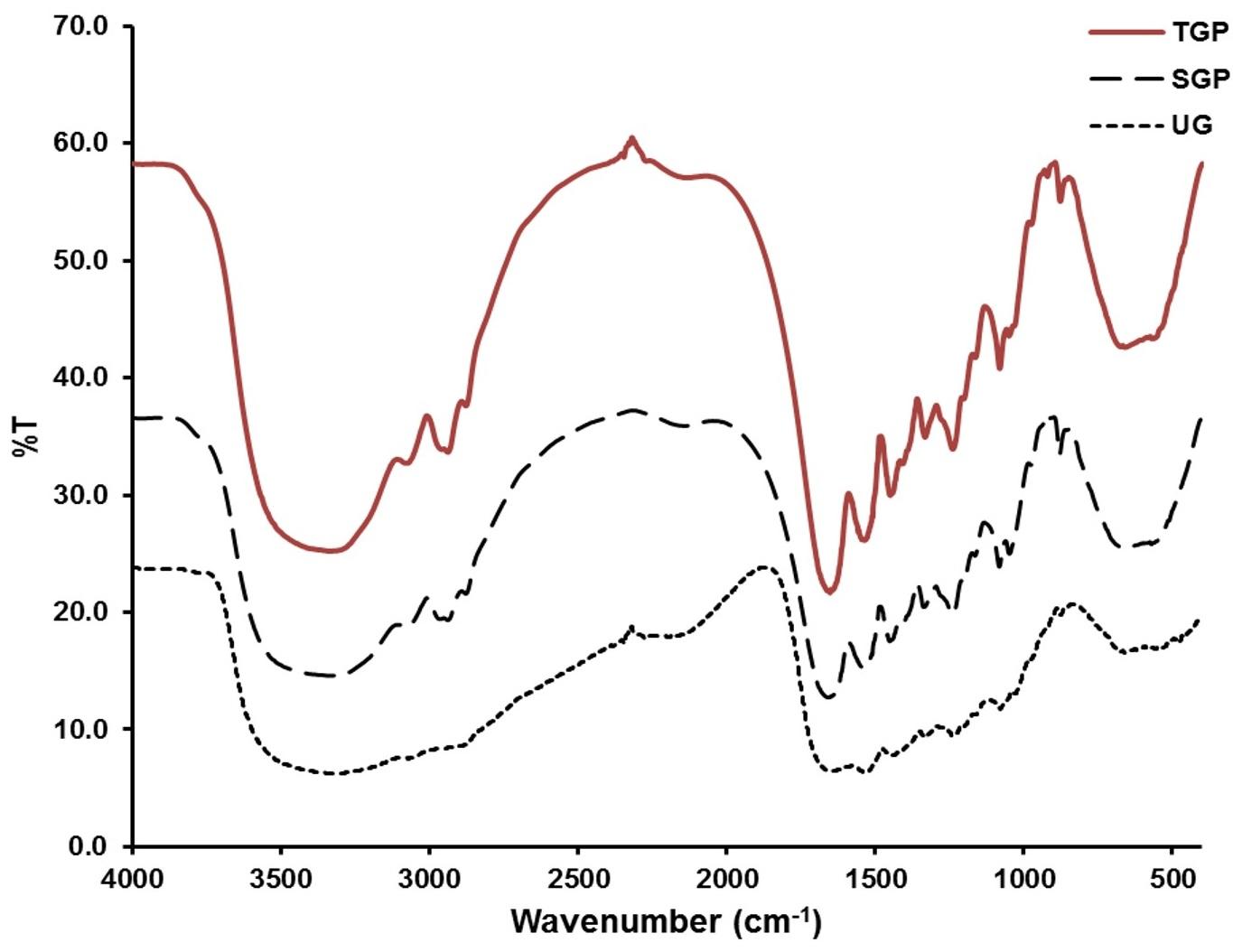
Effect of surfactant concentration to CMC ratio on Zeta potential of gelatin particle
From studying the particle stability by determining the zeta potential on the particle surfaces, it was found that the zeta potential of TGP and SGP measured falls within the range of -30 to -60 millivolts (Figure 3). Zeta potential has long been considered a reliable indicator for evaluating the stability of colloidal systems. In general, particles with zeta potential above +30 mV or below −30 mV are deemed stable [32]. This suggested that gelatin particles were not aggregated because of the negative charge on each particle. The zeta potential of SGP was significantly lower than that of TGP. In addition, the surfactant concentration to CMC ratio showed no statistical differences on the measured zeta potential.
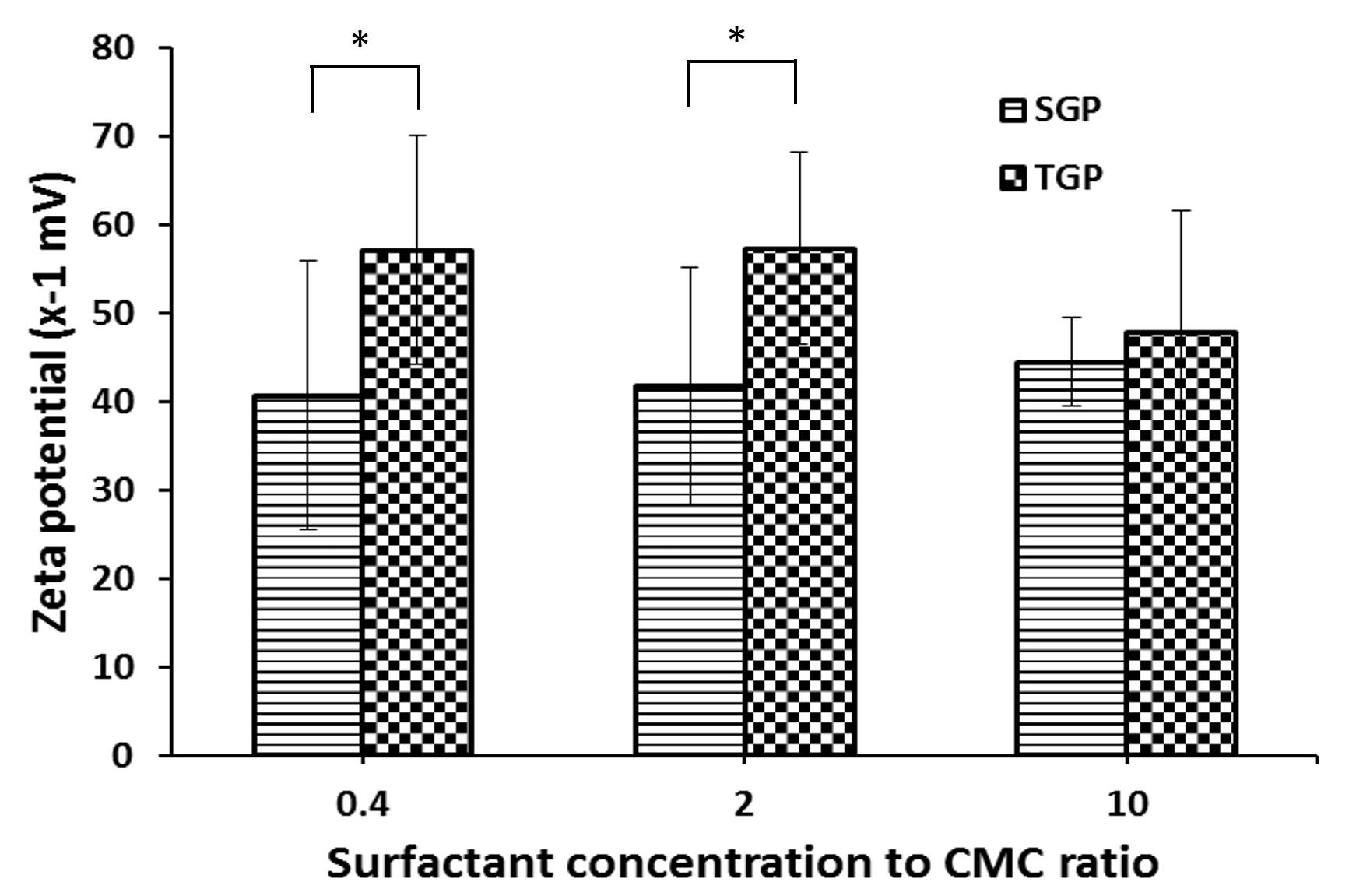
Effect of surfactant concentration to CMC ratio on size of gelatin particle
DLS technique is particularly useful for studying particles in the nanometer to submicron size range, making it suitable for applications such as measuring the size of gelatin particles (Figure 4). After studying the size of TGP prepared at different tween20 concentrations, it was found that the particle sizes were relatively close and did not differ significantly, and they are approximately 95 nm. The prepared particles also exhibited a uniform distribution. For SGP, their sizes ranged between 95-120 nm and increased upon increasing SDS concentration.
The SEM was used to identify and determine the morphology of the gelatin particles. The results showed that SGP and TGP had a round shape with a smooth surface (Figure 5). From SEM images, average diameters of TGP prepared at 0.4, 2, and 10 surfactant concentration to CMC ratio were 84.3, 90.0, and 98.6 nm, respectively, while, average diameters of SGP prepared at 0.4, 2, and 10 surfactant concentration to CMC ratio were 99.0, 101.4, and 120.0 nm, respectively. It is seen that the size of the gelatin particle increased upon increasing the surfactant concentration. The size analyzed by SEM was also the same magnitude as the size detected by DLS reported in the earlier section. However, agglomerated particles were less found in SGP prepared at 10 surfactant concentration to CMC ratio and TGP prepared at two surfactant concentration to CMC ratio. This suggested that these two surfactant formulations were the optimal conditions for preparing stable gelatin particles for further particle degradation and aptamer loading studies.
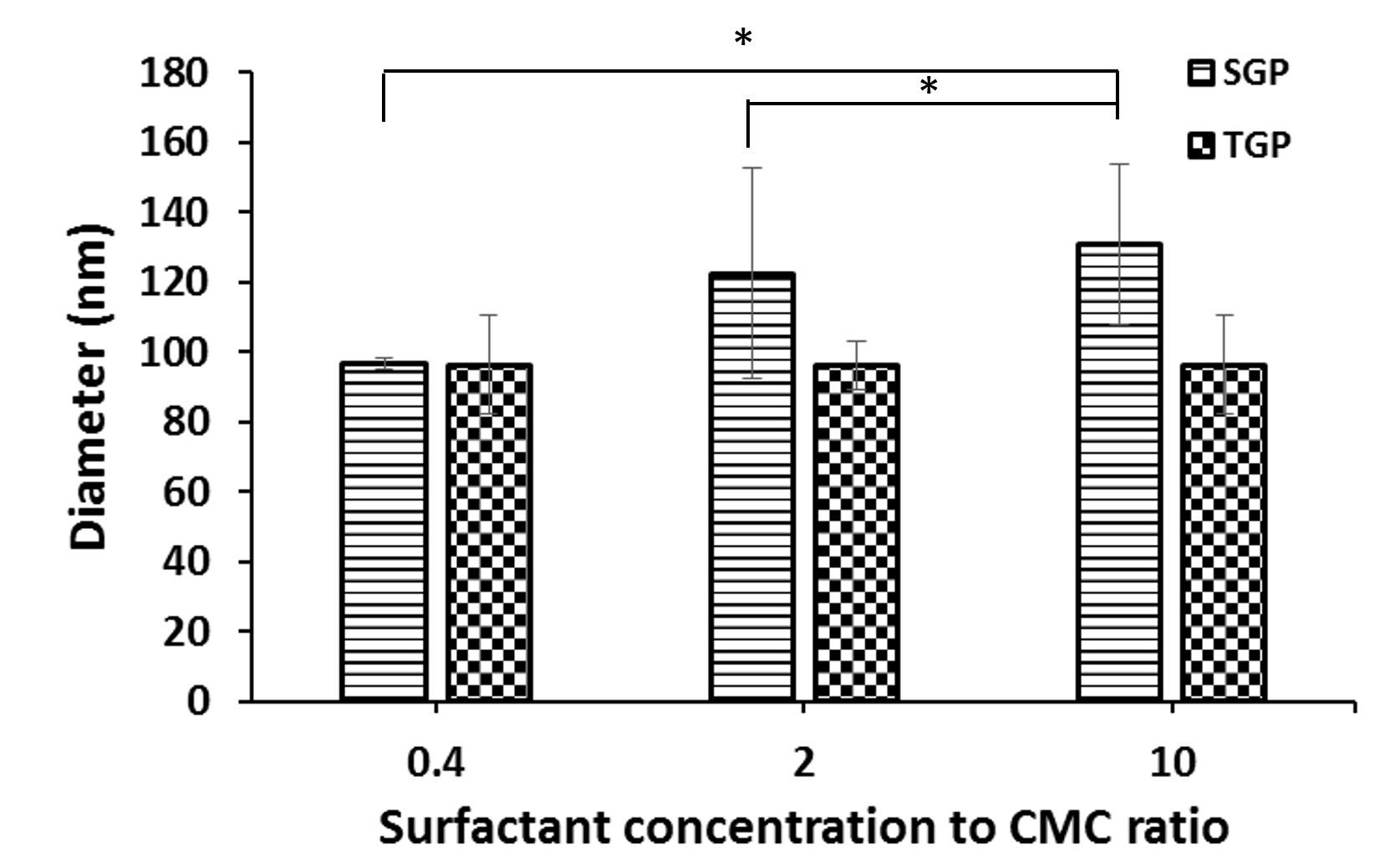
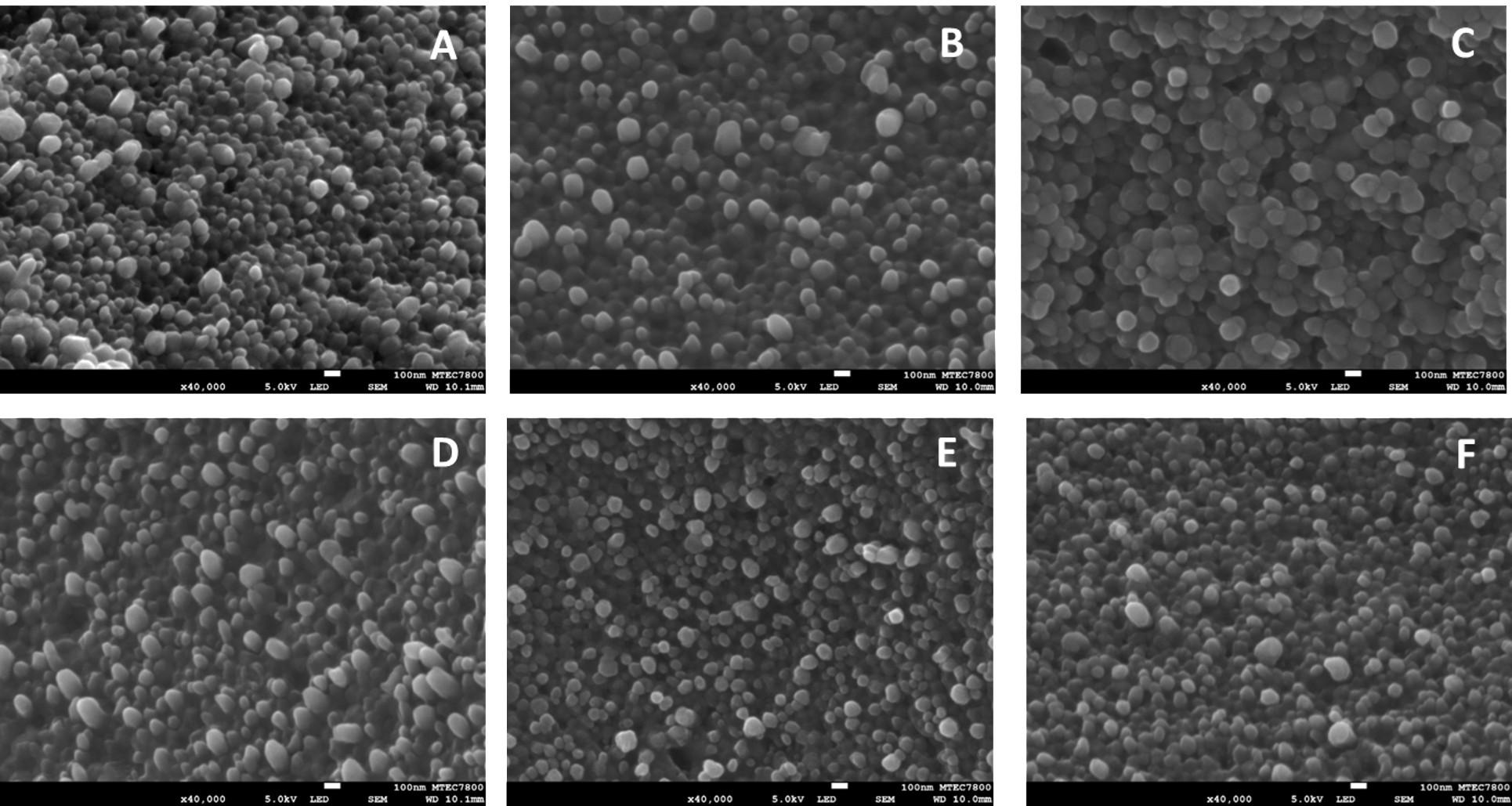
Degradation characteristics of gelatin particle
To further investigate the degradation characteristics of the gelatin particles prepared by using the two different surfactants, a weight loss study in PBS was conducted under ambient conditions. At one hour of incubation, SGP and TGP showed weight losses of 9.33% and 4.67%, respectively (Figure 6). Thereafter, SGP showed a linear dependence between weight loss and time of incubation with overall rates of 0.066 %/hour, which followed zero-order degradation kinetics. Whereas TGP demonstrated its degradation characteristics fitting with first-order kinetics with a degradation rate of 0.96%. As described in the literature, the weight loss kinetics of gelatin-based materials depended on preparation conditions [33]. In addition, within 72 hours of the weight loss study, both SGP and TGP regained their weight over 90 %, indicating the particle stability improvement due to the chemical crosslink reaction compared to the degradation of unmodified gelatin reported in the previous study [34, 35].
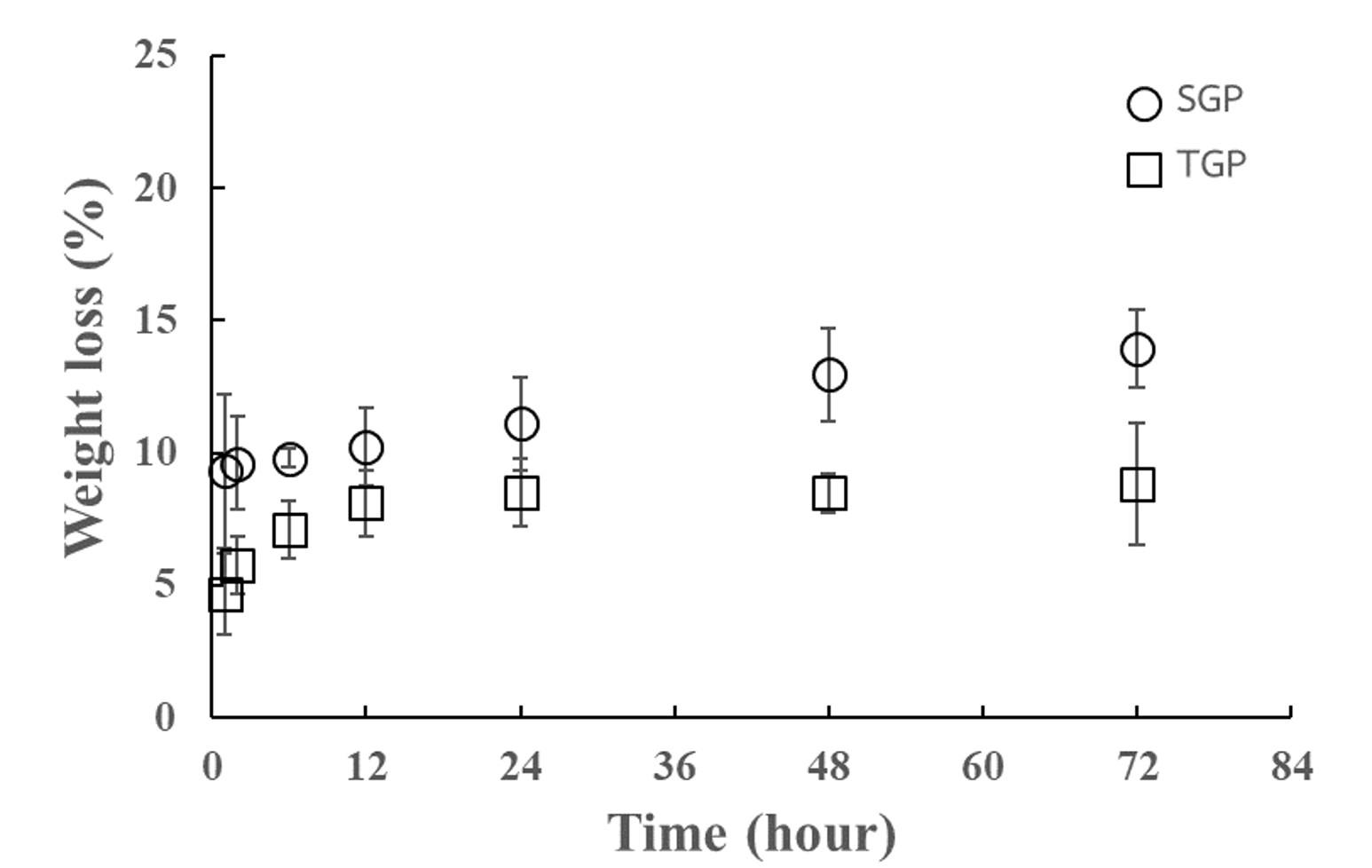
Aptamer release from gelatin particle
Aptamer loading in SGP and TGP was 59.6 and 53.6 mg/g of particles, respectively. The release study was further investigated. Aptamer release from SGP and TGP was tested in neutral (pH 7.4) PBS solutions, and the release of aptamer was recorded for up to 72 hours (Figure 7). After 1 hour, the majority of aptamers were released from the particles: 87% for SGP and 78% for TGP. The cumulative release from SGP reached a plateau after 2 hours, while that from TGP kept constant and maximum level after 1 hour of the release study. The cumulative release level from SGP was higher than that from TGP. The release results did agree with the results from the weight loss study. SGP exhibited higher levels in both degradation and release rate than TGP did. This indicated the impact of surfactants used for particle preparation. Moreover, the abrupt release rates with 2 hours of incubation suggested that the aptamer was successfully loaded onto the surface of the prepared particles. As reported in the literature, SDS and Tween 20 can impact particle size, affecting the loading and release of drugs or bioactive compounds from gelatin particles [36]. Their presence can influence the affinity of molecules for the gelatin matrix, affecting their encapsulation efficiency and release kinetics [37]. Especially, SDS may enhance particle stability depending on the acid-basic environment [38].
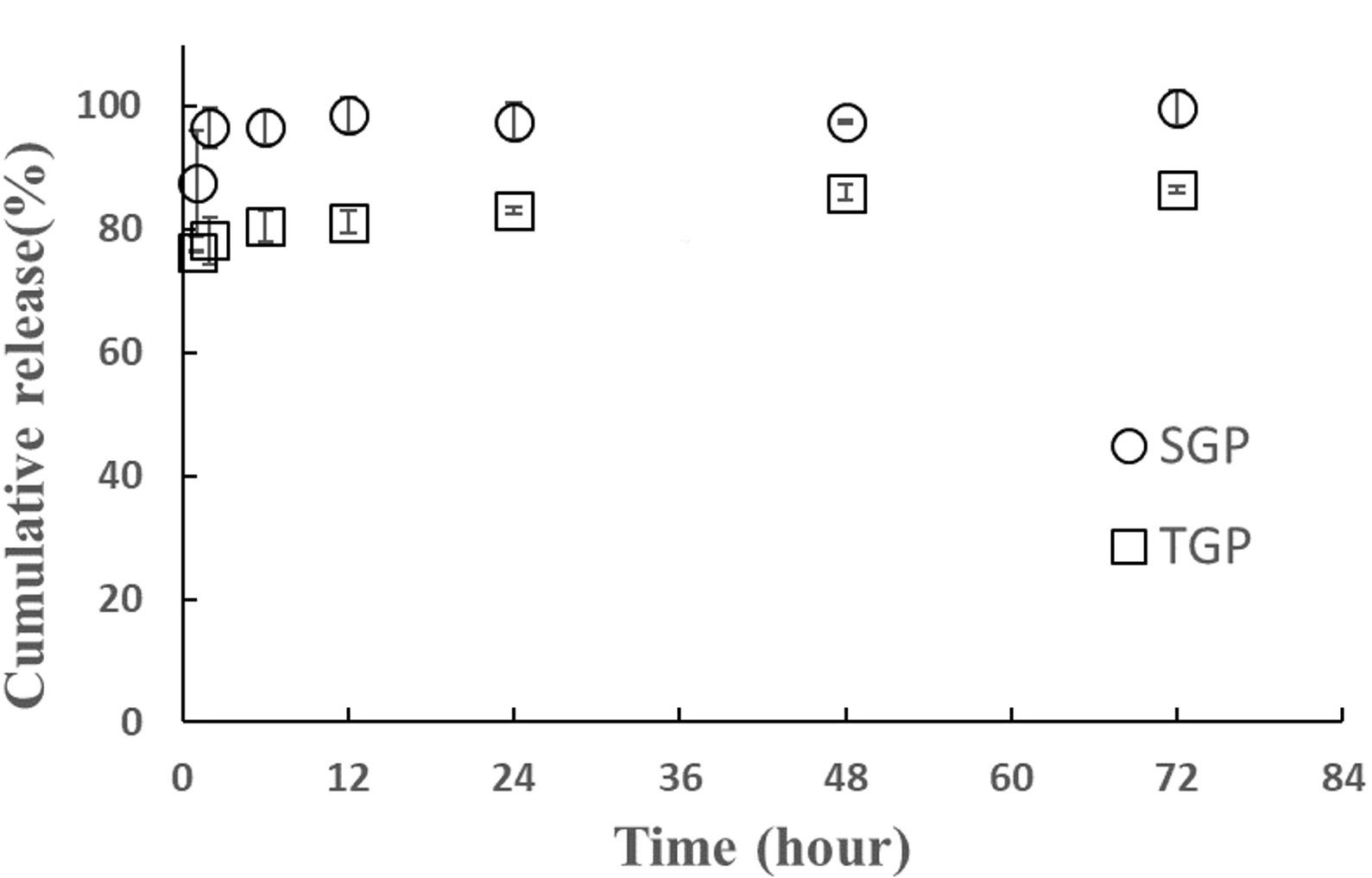
Integrity and stability of released aptamers from gelatin particle
The integrity and stability of released aptamers from gelatin particles are paramount for their efficacy across various applications. Our analysis demonstrated the continuous presence of intact aptamers, evidenced by a consistent single band detected via electrophoresis assay throughout the release period. Notably, each band exhibited comparable fluorescent intensity, as depicted in Figure 8. This indicated the protective role of both SGP and TGP in maintaining aptamer integrity, shielding them from degradation by external factors. Our findings parallel those of a previous long-term storage study involving a nano-formulation incorporating Tween 80 as a co-surfactant for etoposide delivery [39]. This similarity highlighted the significance of surfactants in formulations, as they played a crucial role in promoting emulsification properties. Such protective mechanisms provided by surfactant-modified gelatin particles underscored their potential in preserving the integrity of bioactive molecules, thus enhancing their utility in biomedical applications [40].
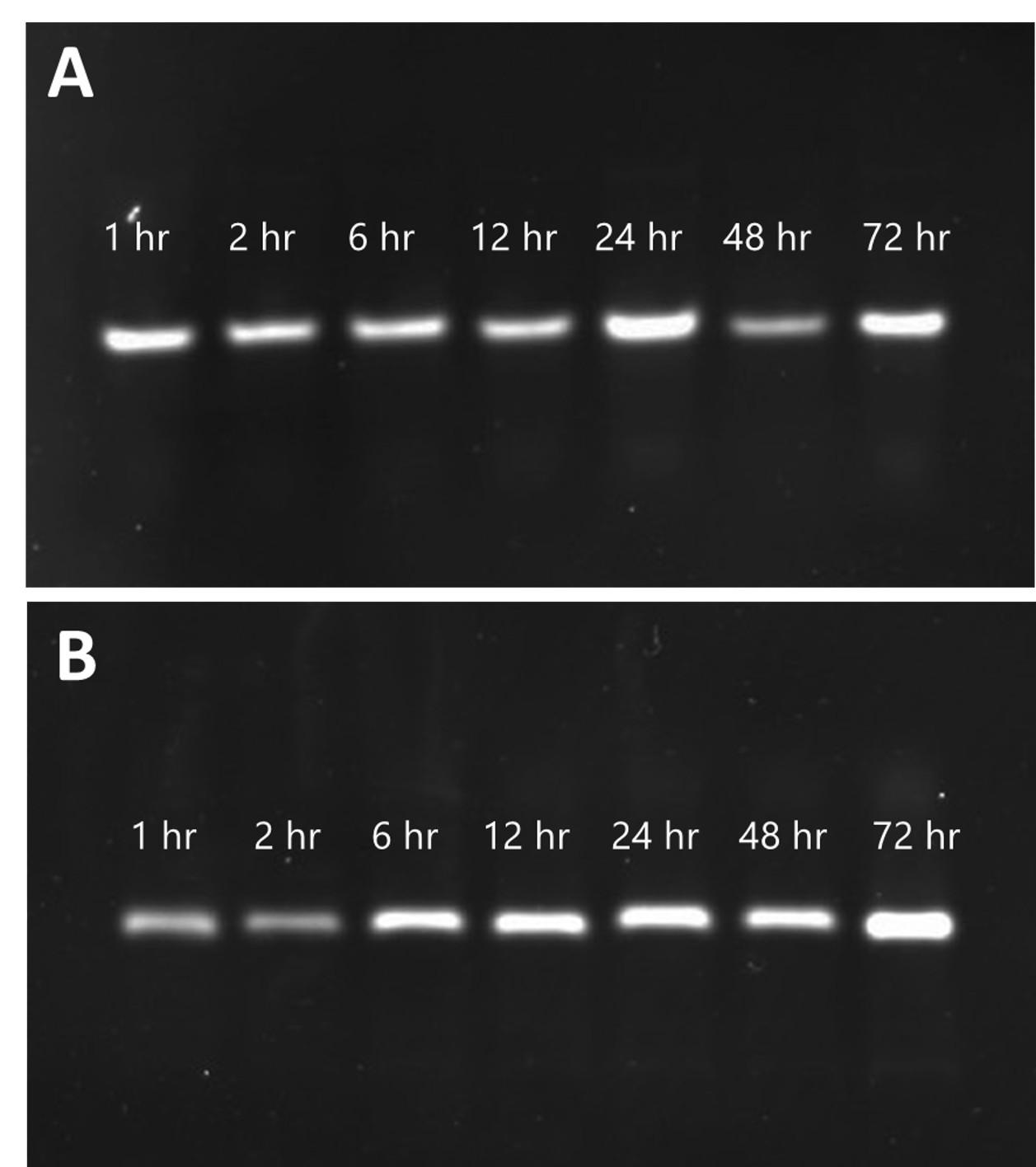
DISCUSSION
While numerous approaches exist, this study focuses on the use of gelatin in drug delivery systems based on particle formulations. Various strategies have been developed for preparing gelatin-based particles, including desolvation, emulsion, chemical crosslinking, nanoprecipitation, and coacervation [41]. Gelatin particles offer a large surface area suitable for functionalization with specific ligands, enabling tailored applications. Additionally, they exhibit rapid drug absorption and release [42]. Recent advancements in gelatin-based drug delivery systems include the following. Gelatin was crosslinked with chitosan using glutaraldehyde to form nanoparticles, which were tested for doxorubicin delivery to H22 cells [43]. Gelatin/alginate particles with basic fibroblast growth factor (bFGF) were prepared via coacervation. These particles effectively stabilized bFGF and enhanced fibroblast viability, migration, and pro-collagen synthesis [44]. Hybrid lipid particles with gelatin, fatty acid, and siRNA were developed. The methacrylate-modified hybrid particles protected siRNA from enzymatic degradation at tumor sites, improving its therapeutic efficacy [45].
Aptamers are promising recognition molecules with advantageous properties, including ease of chemical modification, low immunogenicity, commercial availability, and broad target specificity. Aptamers have been integrated into various applications. Aptamers labeled with chromophores or fluorophores have been used as aptamer beacons for biomolecule monitoring [46]. Alternatively, coupling aptamers with dye-binding assays preserves the aptamer's recognition domain structure by avoiding direct sequence modifications [47]. Aptamers have demonstrated therapeutic potential for diseases such as ocular disorders, hematological conditions, and cancers [48]. In 2004, the FDA approved a VEGF-targeting aptamer for treating Age-Related Macular Degeneration (AMD) [49]. Additionally, a PDGF-BB-targeting aptamer was shown to suppress colon cancer cell proliferation by downregulating the Ras/Raf/MEK/ERK signaling pathway [50].
Although our study highlights the crucial role of surfactant selection and preparation conditions in optimizing gelatin particles for drug delivery applications, several challenges remain. First, the degradation and aptamer release studies were limited to 72 hours, which may not fully capture long-term stability and release dynamics. Extending the study duration could provide deeper insights into sustained release and degradation kinetics. Additionally, aptamer release was primarily assessed in a neutral PBS environment (pH 7.4), whereas physiological conditions vary, such as acidic tumor microenvironments or enzymatic degradation in biological fluids. Evaluating particle behavior under diverse pH and enzymatic conditions would enhance the relevance of the findings for real applications.
CONCLUSIONS
This work elucidated the successful preparation and characterization of gelatin particles, comparing unmodified gelatin with surfactant-modified gelatin particles (SGP and TGP). The ninhydrin assay and FTIR analysis confirmed effective crosslinking between gelatin and glutaraldehyde, resulting in stable particles. Zeta potential measurements indicated that both SGP and TGP remained stable within a range of -30 to -60 mV. DLS and SEM analyses showed that higher surfactant concentrations led to larger particles, measuring between 95 and 120 nm. Weight loss studies revealed that SGP followed zero-order kinetics, while TGP exhibited first-order degradation, with both retaining over 90% of their weight after 72 hours. Aptamer release studies demonstrated a higher release rate for SGP compared to TGP, aligning with their degradation profiles. Lastly, electrophoresis confirmed the integrity of released aptamers, highlighting the protective function of gelatin particles. Overall, these findings underscore the importance of surfactant choice and preparation conditions in tailoring the properties of gelatin particles for various biomedical applications.
ACKNOWLEDGEMENTS
The authors gratefully acknowledge the financial support provided by the Thammasat University Research Fund, Contract No. TUFT 48/2566. KJ received a scholarship from the Faculty of Science and Technology, Thammasat University, and the National Nanotechnology Center (NANOTEC), National Science and Technology Development Agency (NSTDA).
AUTHOR CONTRIBUTIONS
BS and WP were involved in the conception and design of the experiments. WP, PA, and KJ contributed to performing the experiments. WP, PR, and BS analyzed the data. WP and BS contributed to drafting the article. JA, PR, and BS contributed to revising it critically for important intellectual content. All authors made the final approval of the version to be published.
CONFLICTS OF INTEREST
There is no conflict of interest among the authors.
References
- [1]Torchilin VP. Multifunctional, stimuli-sensitive nanoparticulate systems for drug delivery. Nat Rev Drug Discov. 2014;13:813-27.
- [2]Rotkrua P, Lohlamoh W, et al. A molecular hybrid comprising as1411 and pdgf-bb aptamer, cholesterol, and doxorubicin for inhibiting proliferation of sw480 cells. J Mol Recognit. 2021;34.
- [3]Tan HS, Hou N, et al. Cd133 antibody targeted delivery of gold nanostars loading 18820 and docetaxel for multimodal imaging and near-infrared photodynamic/photothermal/chemotherapy against castration resistant prostate cancer. Nanomed-Nanotechnol Biol Med. 2020;27:11.
- [4]Mourtas S, Lazar AN, et al. Multifunctional nanoliposomes with curcumin-lipid derivative and brain targeting functionality with potential applications for alzheimer disease. Eur J Med Chem. 2014;80:175-83.
- [5]Ruan HT, Yao SY, et al. Stapled rap12 peptide ligand of lrp1 for micelles-based multifunctional glioma-targeted drug delivery. Chem Eng J. 2021;403:12.
- [6]Chen ML, Amerigos J, et al. Folate receptor-targeting and reactive oxygen species-responsive liposomal formulation of methotrexate for treatment of rheumatoid arthritis. Pharmaceutics. 2019;11:23.
- [7]Fletcher NL, Houston ZH, et al. Designed multifunctional polymeric nanomedicines: Long-term biodistribution and tumour accumulation of aptamer-targeted nanomaterials. Chem Commun. 2018;54:5.
- [8]Yan XF, Yang Y, et al. Gambogic acid grafted low molecular weight heparin micelles for targeted treatment in a hepatocellular carcinoma model with an enhanced anti-angiogenesis effect. Int J Pharm. 2017;522:110-8.
- [9]Huang SS, Wei SC, et al. Gold nanoparticles modified with self-assembled hybrid monolayer of triblock aptamers as a photoreversible anticoagulant. J Control Release. 2016;221:9-17.
- [10]Tseng YC, Mozumdar S, et al. Lipid-based systemic delivery of sirna. Adv Drug Deliv Rev. 2009;61:721-31.
- [11]Oh EJ, Park K, et al. Target specific and long-acting delivery of protein, peptide, and nucleotide therapeutics using hyaluronic acid derivatives. J Control Release. 2010;141:2-12.
- [12]Islam N, Dmour I, et al. Degradability of chitosan micro/nanoparticles for pulmonary drug delivery. Heliyon. 2019;5:9.
- [13]Segers VFM, Lee RT. Local delivery of proteins and the use of self-assembling peptides. Drug Discov Today. 2007;12:561-8.
- [14]Sun N, Liu M, et al. Chitosan nanofibers for specific capture and nondestructive release of ctcs assisted by pcbma brushes. Small. 2016;12:5090-7.
- [15]Hao XJ, Silva EA, et al. Angiogenic effects of sequential release of vegf-a(165) and pdgf-bb with alginate hydrogels after myocardial infarction. Cardiovascular Research. 2007;75:178-85.
- [16]Venkatesh S, Wower J, et al. Nucleic acid therapeutic carriers with on-demand triggered release. Bioconjugate Chem. 2009;20:1773-82.
- [17]Ma YK, Ge YX, et al. Advancement of multifunctional hybrid nanogel systems: Construction and application in drug co-delivery and imaging technique. Mater Sci Eng C-Mater Biol Appl. 2017;71:1281-92.
- [18]Tungkavet T, Sirivat A, et al. Stress relaxation behavior of (ala-gly-pro-arg-gly-glu-4hyp-gly-pro-) gelatin hydrogels under electric field: Time-electric field superposition. Polymer. 2013;54:2414-21.
- [19]Hudson D, Margaritis A. Biopolymer nanoparticle production for controlled release of biopharmaceuticals. Critical Reviews in Biotechnology. 2014;34:161-79.
- [20]Tungkavet T, Seetapan N, et al. Improvements of electromechanical properties of gelatin hydrogels by blending with nanowire polypyrrole: Effects of electric field and temperature. Polymer International. 2012;61:825-33.
- [21]Ozeki M, Ishii T, et al. Controlled release of hepatocyte growth factor from gelatin hydrogels based on hydrogel degradation. Journal of Drug Targeting. 2001;9:461-71.
- [22]Fukunaka Y, Iwanaga K, et al. Controlled release of plasmid DNA from cationized gelatin hydrogels based on hydrogel degradation. Journal of Controlled Release. 2002;80:333-43.
- [23]Sun SG. Technology evaluation: Selex, gilead sciences inc. Current Opinion in Molecular Therapeutics. 2000;2:100-5.
- [24]Proske D, Blank M, et al. Aptamers--basic research, drug development, and clinical applications. Appl Microbiol Biotechnol. 2005;69:367-74.
- [25]Soontornworajit B, Wang Y. Nucleic acid aptamers for clinical diagnosis: Cell detection and molecular imaging. Anal Bioanal Chem. 2011;399:1591-9.
- [26]Zhan Y, Ma W, et al. DNA-based nanomedicine with targeting and enhancement of therapeutic efficacy of breast cancer cells. ACS Applied Materials and Interfaces. 2019;11:15354-65.
- [27]Yazdian-Robati R, Bayat P, et al. Therapeutic applications of as1411 aptamer, an update review. Int J Biol Macromol. 2020;155:1420-31.
- [28]Soliman AG, Mahmoud B, et al. Optimized synthesis characterization and protective activity of quercetin and quercetin–chitosan nanoformula against cardiotoxicity that was induced in male wister rats via anticancer agent: Doxorubicin. Cancer Nanotechnology. 2023;14.
- [29]Soontornworajit B, Zhou J, et al. Aptamer-functionalized in situ injectable hydrogel for controlled protein release. Biomacromolecules. 2010;11:2724-30.
- [30]Bahoor A, Ahmadi R, et al. Synthesis and evaluation of cross-linked gelatin nanoparticles for controlled release of an anti-diabetic drug: Gliclazide. Inorganic Chemistry Communications. 2023;154.
- [31]Dilruba Öznur KG, Ayşe Pınar TD. Statistical evaluation of biocompatibility and biodegradability of chitosan/gelatin hydrogels for wound-dressing applications. Polymer Bulletin. 2024;81:1563-96.
- [32]Zhang W, Shen H, et al. Preparation of type-a gelatin/poly-γ-glutamic acid nanoparticles for enhancing the stability and bioavailability of (-)-epigallocatechin gallate. Foods. 2023;12.
- [33]Santoro M, Tatara AM, et al. Gelatin carriers for drug and cell delivery in tissue engineering. J Control Release. 2014;190:210-8.
- [34]Aljaber MB, Verisqa F, et al. Influence of gelatin source and bloom number on gelatin methacryloyl hydrogels mechanical and biological properties for muscle regeneration. Biomolecules. 2023;13.
- [35]Soontornworajit B, Kerdsiri K, et al. Aptamer-gelatin composite material for prolonging pdgf-bb release. Songklanakarin Journal of Science and Technology. 2020;42:180-7.
- [36]Vaziri A, Warburton B. Some preparative variables influencing the properties of w/o/w multiple emulsions. Journal of Microencapsulation. 1994;11:649-56.
- [37]Özyildiz F, Karagönlü S, et al. Micro-encapsulation of ozonated red pepper seed oil with antimicrobial activity and application to nonwoven fabric. Letters in Applied Microbiology. 2013;56:168-79.
- [38]Zhang T, Ding M, et al. Droplet and creaming stability of fish oil-loaded gelatin/surfactant-stabilized emulsions depends on both the adsorption ways of emulsifiers and the adjusted ph. Food Science and Human Wellness. 2020;9:280-8.
- [39]Ma X, Wang T, et al. Formulation and physicochemical and biological characterization of etoposide-loaded submicron emulsions with biosurfactant of sophorolipids. AAPS PharmSciTech. 2022;23:181.
- [40]Mishra V, Nayak P, et al. Orally administered self-emulsifying drug delivery system in disease management: Advancement and patents. Expert Opin Drug Deliv. 2021;18:315-32.
- [41]Nitta SK, Numata K. Biopolymer-based nanoparticles for drug/gene delivery and tissue engineering. International Journal of Molecular Sciences. 2013;14:1629-54.
- [42]Aljabali AAA, Rezigue M, et al. Protein-based nanomaterials: A new tool for targeted drug delivery. Therapeutic Delivery. 2022;13:321-38.
- [43]Li K, Zhou D, et al. Size-transformable gelatin/nanochitosan/doxorubicin nanoparticles with sequentially triggered drug release for anticancer therapy. Colloids and Surfaces B: Biointerfaces. 2022;220.
- [44]Lee J, Ban E, et al. Stability enhancement of freeze-dried gelatin/alginate coacervates for bfgf delivery. Pharmaceutics. 2022;14.
- [45]Lei S, Gao Y, et al. Dual-rna controlled delivery system inhibited tumor growth by apoptosis induction and tme activation. J Control Release. 2022;344:97-112.
- [46]Hamaguchi N, Ellington A, et al. Aptamer beacons for the direct detection of proteins. Analytical Biochemistry. 2001;294:126-31.
- [47]Stojanovic MN, Kolpashchikov DM. Modular aptameric sensors. J Am Chem Soc. 2004;126:9266-70.
- [48]Keefe AD, Schaub RG. Aptamers as candidate therapeutics for cardiovascular indications. Current Opinion in Pharmacology. 2008;8:147-52.
- [49]Keefe AD, Pai S, et al. Aptamers as therapeutics. Nat Rev Drug Discov. 2010;9:537-50.
- [50]Sae-Lim S, Soontornworajit B, et al. Inhibition of colorectal cancer cell proliferation by regulating platelet-derived growth factor b signaling with a DNA aptamer. Asian Pac J Cancer Prev. 2019;20:487-94.