Optimization of production and partial characterization of cellulase and protease enzymes from Aeromonas hydrophila ASM-S32
Abstract
Microbial hydrolytic enzymes, especially cellulase and protease, are widely used in industrial processes due to their low cost, large productivity, chemical stability, environmental protection, plasticity and vast availability. But existing cellulase and protease enzymes are not capable enough to fulfill the industrial demands due to the lack of adapting operational conditions. So, the present study focused on extracting enzymes from microbial sources to overcome the problems. 260 isolated bacterial strains from different areas of Sylhet, Bangladesh, were screened for their cellulolytic and proteolytic activities using carboxymethylcellulose (CMC) and skim milk agar respectively. Among them 6 cellulolytic and 15 proteolytic bacteria were initially identified. Based on the capability to degrade CMC and skim milk, strain S32 was found to be the most potential among the isolates. Biochemical tests and molecular identification revealed that S32 is a strain of Aeromonas hydrophila and was later named as Aeromonas hydrophila ASM-S32. Maximum cellulase production by the strain A. hydrophila ASM-S32 was obtained after 18 hours (h) of incubation in a fermentation medium with an initial pH of 6.5 at 37°C and that was 4-fold higher as compared to unoptimized conditions. Maximum cellulase activity was observed at 60°C with a pH of 6.5 in presence of Ca2+ metal ion. In case of protease, optimum enzyme production was observed after 24 h of incubation with an initial pH of 8.5 at 37°C and protease production was increased by 1.2-folds when optimized conditions were used. Maximum protease activity resulted from pH 6.0, at 70°C and in presence of Cu2+ ion.
INTRODUCTION
Global attention has currently been attracted by enzymes due to their wide range of industrial applications in many fields including organic synthesis, clinical analysis, pharmaceuticals, detergents, food production and fermentation [1]. Consequently, the uses of harsh chemicals in various industrial processes are gradually replaced by enzymes [2]. As enzymes work under moderate conditions, such as warm temperatures and neutral pH, they reduce energy consumption by eliminating the need to maintain extreme environments. The reaction specificity of the enzymes lead to minimization of the production of by-products and thereby the application of enzymes offer minimal risk to the environment [2]. Approximately 80% of all industrial enzymes are hydrolytic in nature and are used for depolymerization of natural substances [3].
Hydrolytic enzymes such as cellulases have been commercially available for more than 30 years, and these enzymes have represented a target for both academic as well as industrial research [4, 5]. Cellulose is considered as one of the most important sources of carbon on this planet and its annual biosynthesis by both higher plants and marine algae occurs in many tons per annum. Moreover, numerous agricultural residues generated due to diverse agricultural practices and food processing such as rice straw, yam peels, cassava peels, banana peels represent one of the most important energy resources. The major components of these are cellulose and hemicellulose (75-80%) while lignin constitutes only 14%. Accumulation of these agricultural residues causes deterioration of the environment in each year and huge loss of potentially valuable nutritional constituents which when processed could yield food, feed, fuel, chemicals and minerals. In this case, cellulases can play an important role in different industries; e.g., food processing, animal feed production, pulp and paper production, detergent and textile industry [6]. Bioconversion of biomass to biofuel is also dependent on the catalytic activity of cellulase [5].
Protease is another demandable hydrolytic enzyme which executes a large variety of functions and has important biotechnological applications. Proteases represent one of the three largest groups of industrial enzymes and find application in detergents, leather industry, food industry, pharmaceutical industry and bioremediation processes [7]. Proteases are widespread in nature and due to their key role in biological processes and in the life-cycle of many pathogens, have found immense importance in many industrial sectors. Microbes serve as a preferred source of these enzymes because of their rapid growth, the limited space required for their cultivation and the ease with which they can be genetically manipulated to generate new enzymes with altered properties that are desirable for their various applications [8]. Most of the available proteases produced commercially are of microbial origin [9]. As cellulases and proteases have numerous industrial applications, the demands for more stable, highly active and specific enzymes are growing rapidly.
The present study was aimed to screen potential microorganisms from various sites of North Eastern part of Bangladesh for obtaining both protease and cellulase enzymes that is able to satisfy the present demands. The research work describes the effects of culture conditions on cellulase and protease production in batch experiments in shake flasks and under controlled conditions in a laboratory incubator. In this study, strategies were applied to optimize the production conditions. In addition, effects of temperature, pH and metal ions on the activity of both the enzymes were evaluated.
MATERIALS AND METHODS
Isolation of bacteria
Samples were collected from different dumping sites of Sylhet region in Bangladesh. Samples were then enriched separately for both cellulolytic and proteolytic bacteria by culturing in nutrient broth along with 1% carboxymethylcellulose (CMC) and casein respectively. After 3 days incubation at 37°C and 120 rpm, single colonies were isolated by spread plate method in nutrient agar.
Screening of cellulolytic bacteria
Isolates were grown in 10 ml of nutrient broth for 24 h at 120 rpm and 37°C. Slow growing isolates were left to incubate for an additional 24 h. Resulting broth cultures were tested for cellulase activity via the Gram’s iodine method [10]. In this method, 5μl of each broth culture was singly dropped onto a petri dish containing CMC agar and then incubated for 48 h at 37°C. The CMC agar plates with the isolates were then stained with Gram’s iodine solution (2.0g KI and 1.0g I, per 300 ml ddH2O) to visualize the cellulase activity. This solution stains the agar containing CMC brown and leaves areas without CMC clear, described here as halos. The halo diameters were measured using a ruler for a semi-qualitative comparison of cellulase activity among the isolates. The halo measurement was used to identify comparatively better CMC degrading bacteria [11].
Screening of proteolytic bacteria
Isolates were grown in 10 ml of nutrient broth for 24 h at 120 rpm and 37°C. Slow growing isolates were left to incubate for an additional 24 h. Broth cultures were tested for protease activity by singly dropping 5μl of each broth culture onto a petri-dish containing skim milk agar (SMA) and then incubated for 24 h at 37°C. Bacterial isolates that were able to utilize skim milk form clear zone around the single droplets of bacterial culture. The zone diameters were measured using a ruler for a semi-qualitative comparison of proteolytic activity among the isolates. The zone diameter measurement was used to compare proteolytic bacteria.
Cultural characteristics and biochemical studies
The colony characteristics such as: size, shape, colour of bacterial colonies grown on agar medium was recorded. Morphological characters of the isolates such as shape and size were observed after Gram’s staining with a compound light microscope at 1000x magnification.
Several biochemical tests were performed for the confirmation of the genus of the isolate. The biochemical tests performed were Voge’s Proskauer (VP) test, methyl red (MR) test, catalase test, glucose fermentation tests, indole test and citrate utilization test.
Molecular identification of the selected bacterial isolate
Molecular identification of the bacterial isolate S32 was performed by 16s rDNA sequence analysis by Invent Technologies Ltd., Bangladesh. Then the obtained sequences were compared with those of 16S rDNA deposited at GenBank by using BLAST program (http://blast.ncbi.nlm.nih.gov/Blast.cgi) and closely related sequences were multiple aligned using ClustalX 2.0 to identify organism with maximum similarity. Isolate S32 was found to have about 96% similarity with Aeromonas hydrophila. A phylogenetic tree was constructed using MEGA 5.2 software.
Optimization of pH and temperature for the growth of seed culture
Selected isolate was grown in nutrient broth medium at different pH (6.0, 6.5, 7.0, 7.5, 8 and 8.5) to identify the optimum pH for its growth. Optimum temperature for the growth of the selected isolate was identified by culturing it in nutrient broth medium at different temperatures (30°, 33°, 35°, 37° and 40°C).
Production of crude cellulase enzyme
Seed culture was prepared in nutrient broth with an incubation time of 24 h at 37°C and 120 rpm. For the production of cellulase from bacteria, Czapek’s basal media (K2HPO4 0.1%, MgSO4.7H2O 0.05%, KNO3 0.3%, FeSO4.5H2O 0.001%, (NH4)2SO4 0.15%, Yeast extract 0.12%, CMC (carboxymethylcellulose) 1.0%) was used as basal medium. The initial pH of the medium was adjusted to 7.0 with 0.1N NaOH and 0.1N HCl. Conical flasks (250 ml) containing 90 ml of medium were inoculated with 10 ml of an overnight seed culture and incubated at 37ºC in a rotary shaker incubator at 120 rpm for 24 h.
Assessment of cellulase activity
Cellulase activity was assayed using Miller method in which 1.5 ml reaction mixture containing 0.5 ml of diluted enzyme solution and 1 ml of 2% CMC suspension in 0.05 M citrate buffer (pH 6) was used. The reaction mixture was incubated at 45ºC for 20 minutes. Following incubation, the reaction was stopped by addition of 3 ml of di-nitrosalicylic acid (DNS) and boiling for 10 minutes at 99ºC. After cooling, optical density (OD) was measured at 540 nm by spectrophotometer (PG instrument LTD T80, UK). One unit (U) of enzyme activity was defined as the amount of enzyme that released 1 µmol of glucose per minute under the assay conditions.
Production of crude protease enzyme
Seed culture was prepared in nutrient broth with an incubation time of 24 h at 37°C and 120 rpm. The enzyme production was carried out in the basal medium (Glucose 10.0g, Peptone 10.0g, K2HPO4 1.0g, MgSO4 0.2g, Na2CO3 5.0g, Distilled water 1000 ml). The initial pH of the medium was adjusted to 7.0 with 0.1N NaOH and 0.1N HCl. The medium was sterilized by autoclaving at 15 lbs /inch2 pressure (121ºC) for 15 minutes. Conical flasks (250) containing 90 ml of medium were inoculated with 10 ml of an overnight seed culture and incubated at 37ºC in a rotary shaker incubator at 120 rpm for 24 h.
Protease activity assay
Protease activity was determined by modified Anson method [12] using 1% casein as substrate. 0.2 ml of enzyme solution was added to 0.8 ml of substrate solution (1% V/V, casein with 50 mM Glycine-NaOH buffer, pH 10.0) and incubated at 50˚C for 20 minutes independently with respective controls. The reaction was stopped by adding 1 ml of 10 % TCA followed by 10 min holding at room temperature which was then centrifuged at 8000 rpm for 15 min at 4°C.
1 ml of supernatant was added to 3 ml of 0.4M Na2CO3 solution. Then 0.5 ml of Folin reagent was immediately added to each tube, vortexed and left for 30 min at room temperature. This provides coloration equivalent, measured at OD660 nm, to 1 μmol of tyrosine, in the presence of the Folin-Ciocalteau reagent by using a tyrosine standard curve [13]. The absorbance was measured at 660 nm. The protease activity was expressed as the difference of absorbance at 660 nm between the control and the test sample.
Optimization of production period for cellulase and protease production
Cultivation period is an important parameter for enzyme production. In this study, to determine the optimum cultivation period for maximum cellulase and protease production, the seed cultures were inoculated in the respective basal media and incubated at 37°C and 120 rpm. Experiment was carried out up to 30 hours and enzyme activities were measured at 6 hours intervals according to the previously mentioned methods.
Optimization of pH for cellulase and protease production
To observe the effect of different initial pH on the production of cellulase by the selected bacterial isolate, media were adjusted to pH 6.0, 6.5, 7.0, 7.5, and 8.0 in different flasks using 0.05M buffer (phosphate buffer for 6.0 to 8.0 pH). In case of protease enzyme, cultivation was carried out in the medium of different initial pH values e.g. 6.0, 6.5, 7.0, 7.5, 8.0, 8.5, 9.0, 9.5, 10.0 and 10.5. The pH of the media was adjusted with different suitable buffers (phosphate buffer for 6.0 to 8.0 pH and Glycine-NaOH buffer for 8.5 to 10.5 pH). The production media were incubated at 37°C and 120 rpm. Cellulase and protease activities were determined at different pH according to the methods described previously.
Optimization of temperature for cellulase and protease production
Production medium at pH 6.5 was inoculated with overnight grown selected bacterial strain. The broth was incubated at different temperatures 30°, 35°, 37°, 40°C for 18 hours. At the end of incubation period, cellulase activities were measured by the previously mentioned method. In order to assess the optimum temperature for protease production by the selected isolate, the preparations were cultivated at different temperatures e.g. 30°C, 35°C, 37°C, 40°C, at 120 rpm. The pH of the media was adjusted to 8.5. After 24 hours of incubation, the activities of the crude enzyme solutions were determined according to the method described previously.
Optimization of pH for cellulase and protease assay condition
The effect of pH on cellulase activity was evaluated by carrying out cellulase activity assay in pH 6.0, 6.5, 7.0, 7.5, 8.0 and 8.5. These conditions were adjusted by using different buffers (phosphate buffer for 6.0 to 8.0 pH and Glycine-NaOH buffer for 8.5 pH). Cellulase activities were measured for individual pH condition by the previously stated method. On the other hand, protease activity assay was carried out in pH 6.0, 6.5, 7.0, 7.5, 8.0, 8.5, 9.0, 9.5, 10.0 and 10.5 using similar buffers (phosphate buffer for 6.0 to 8.0 pH and Glycine-NaOH buffer for 8.5 to 10.5 pH). Protease activities were measured for individual pH condition by the previously stated method.
Optimization of temperature for cellulase and protease assay condition
Cellulase activity assays were conducted at 45°, 50°, 55°, 60°, 65° and 70°C reaction temperatures. Reaction pH was adjusted to pH 6.5. Conversely, protease activity assays were conducted at 45°, 50°, 55°, 60°, 65°,70° and 75°C reaction temperatures. Reaction pH was adjusted to pH 6. Previously stated methods were used for measuring cellulase and protease activities.
Optimization of metal ions for cellulase and protease assay condition
Different metal salts at a concentration of 10 mM were dissolved in phosphate buffer. Cellulase and protease activities were measured for reaction mixtures containing one of these metal ions. Metal salts that were used for determining the effects of respective metal ions were Mg2+, Ca2+, Cu2+, Zn2+, K+.
Statistical analysis
Data analysis was performed using SPSS software version10 (Chicago, USA).The results were presented as mean ± SE. In case of effects of metal ions on the activity of cellulase and protease enzymes, analysis of variance (ANOVA) was performed using SPSS Version 25 to investigate the significance of the treatment effect means.
RESULTS
Screening and identification of cellulolytic and proteolytic bacterial isolates
The appearance of clear halos around the bacterial culture drops confirms bacterial cellulase activity. Among 260 bacterial isolates, only 6 isolates produced halos around their culture drops (Figure 1a). Bacterial isolates that were able to utilize skim milk formed clear zone around the droplets of their culture. Among 260 bacterial isolates, 15 bacterial isolates produced clear zone around their culture drops (Figure 1b). Interestingly, 6 bacterial isolates were found to have both cellulolytic and proteolytic activities. Moreover, among these 6 bacterial isolates S32 revealed as the best cellulolytic and proteolytic bacteria. Comparison of the cellulolytic and proteolytic activity of different bacterial isolates has been shown in Figure 2.
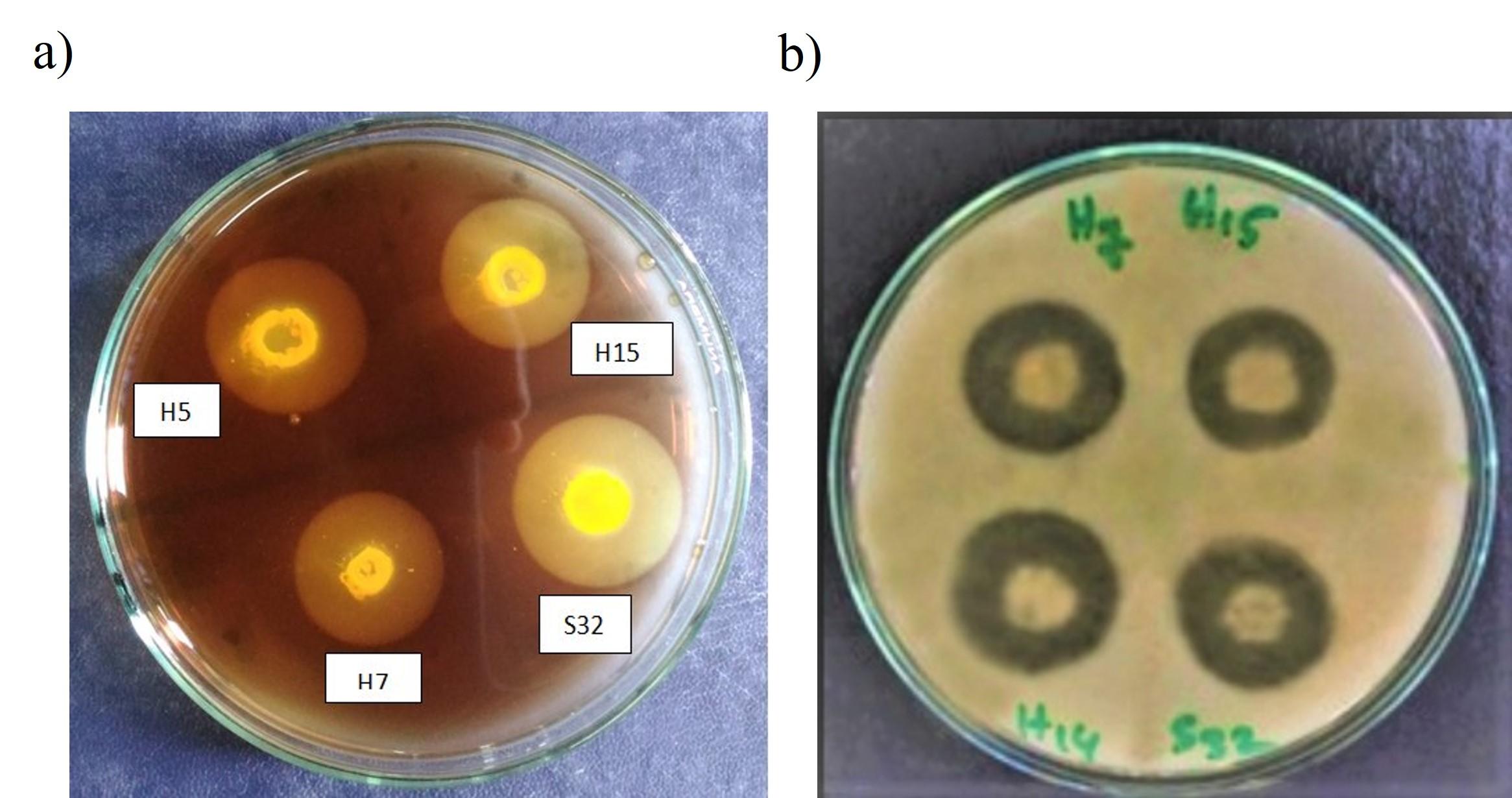
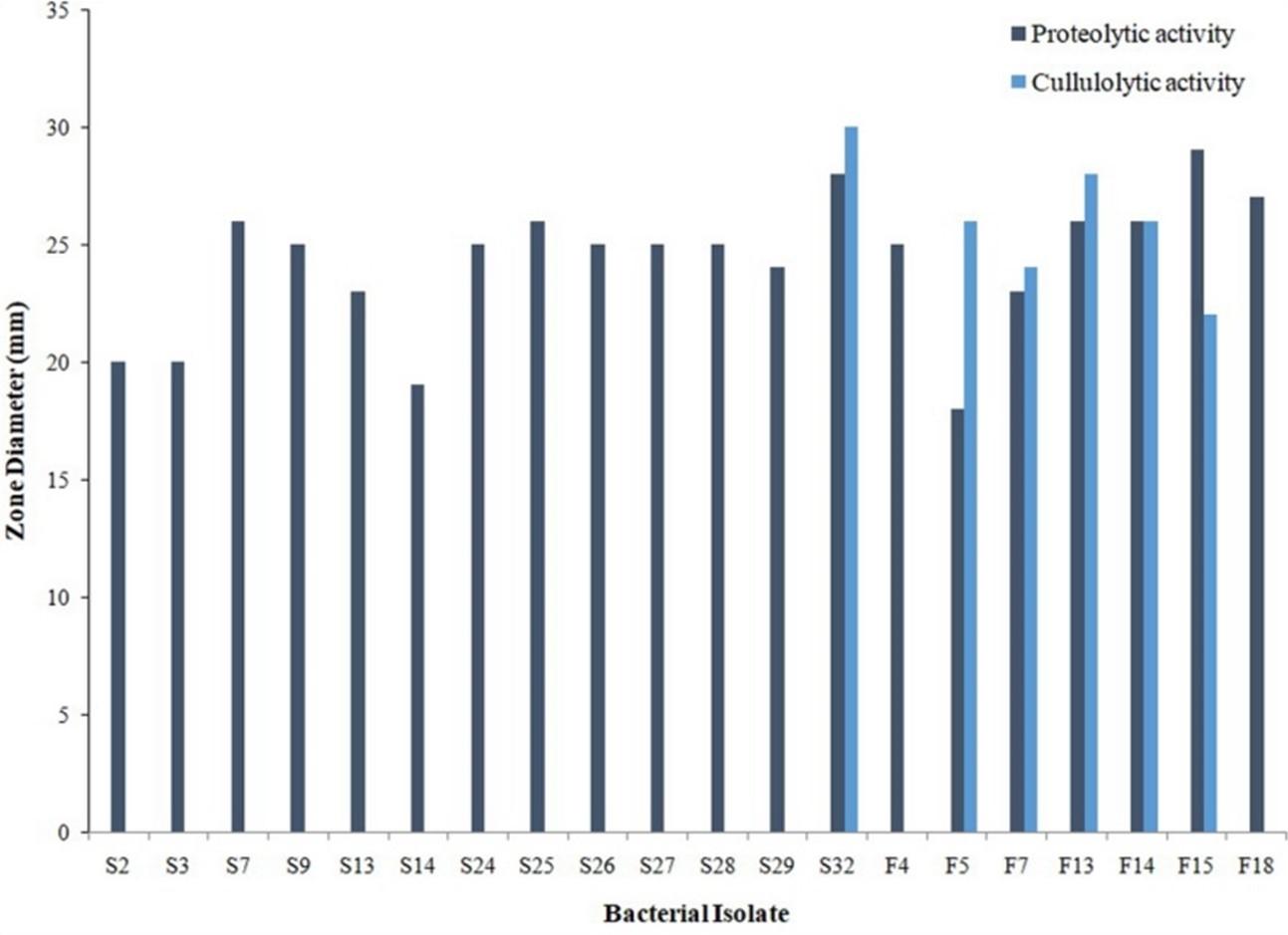
Cultural characteristics and biochemical studies
The bacterial isolate was Gram negative and rod shaped. The diameter of its smooth, convex, round, opaque colonies was 2-3mm. Results of several biochemical tests were Voges-Proskauer (VP) test negative, methyl red (MR) test positive, catalase test negative, glucose fermentation tests positive, indole test positive and citrate utilization test negative (data not shown).
Molecular identification of the selected bacterial isolate
16s rDNA sequence of bacterial isolate S32 was 1422 bp long which is shown in Figure 3. Phylogenetic Tree revealed that S32 exhibited maximum sequence similarity with Aeromonas hydrophila strain_IR32 (Figure 4).
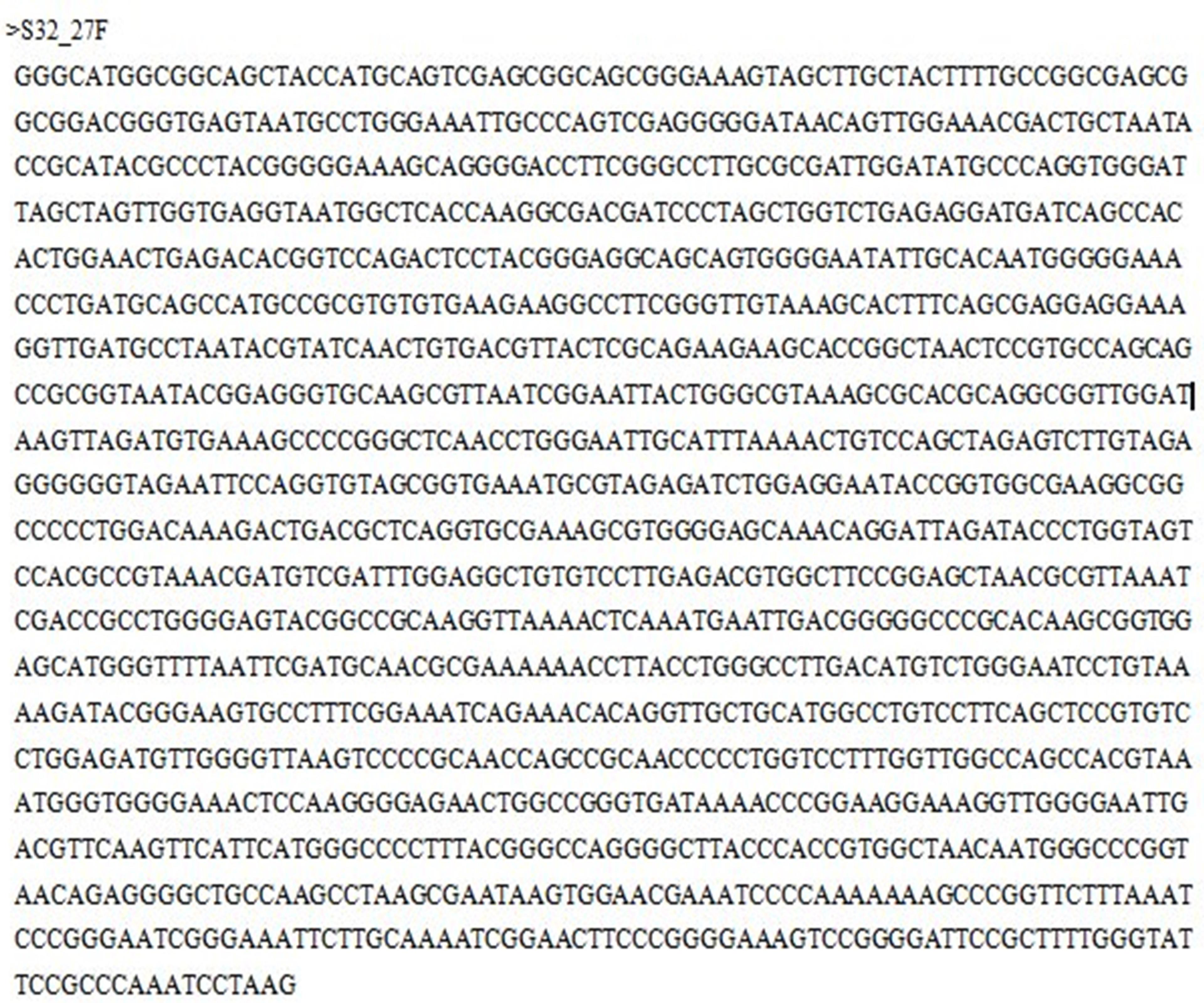
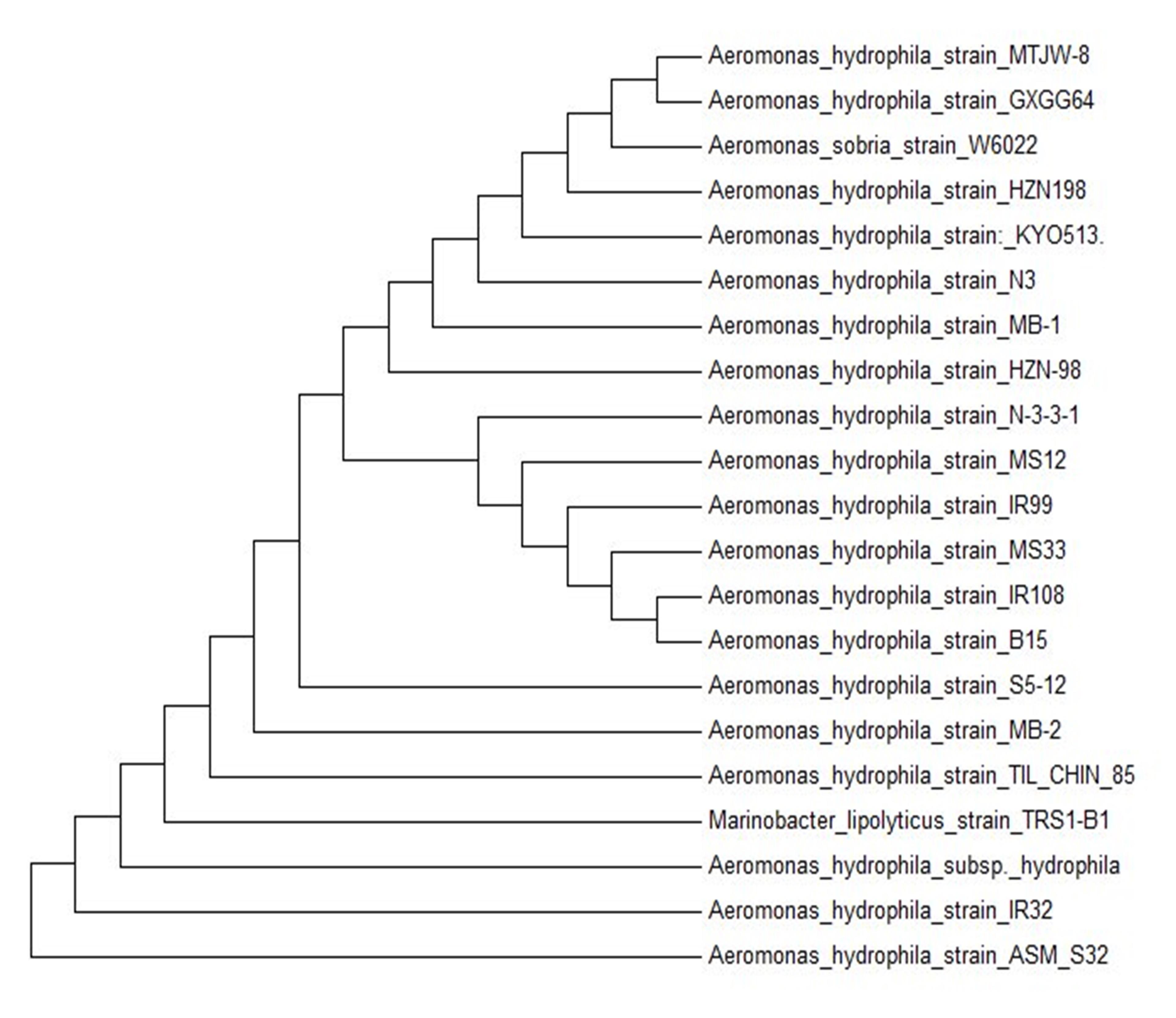
Optimization of Cellulase and Protease Production
Cellulase activity of the bacterial isolate was maximum with 18 hours of production period (Figure 5). Among the different pH and temperatures with pH 6.5 (Figure 6) and 37°C temperature (Figure 7) maximum cellulase activity was observed.
In case of protease maximum activity was observed with 24 hours of production period (Figure 8). The bacterial isolate provide maximum protease activity with pH 8.5 (Figure 9) and 37°C temperature (Figure 10).
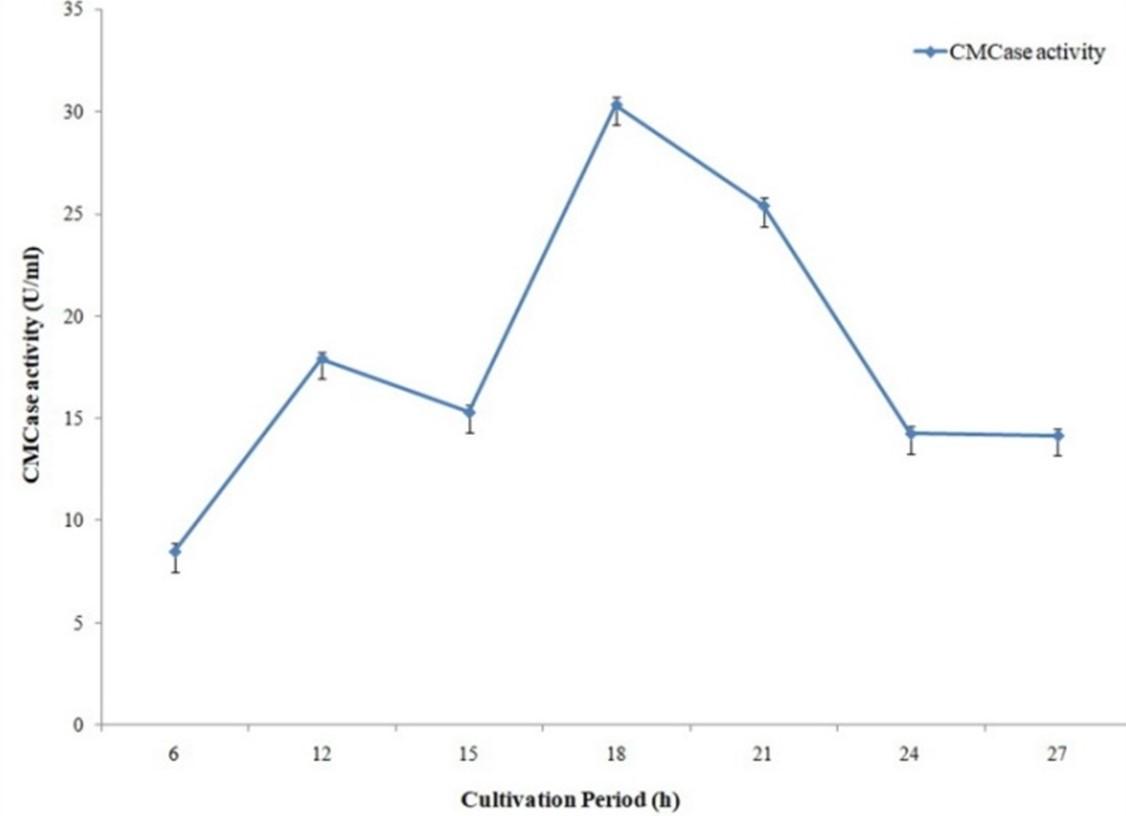
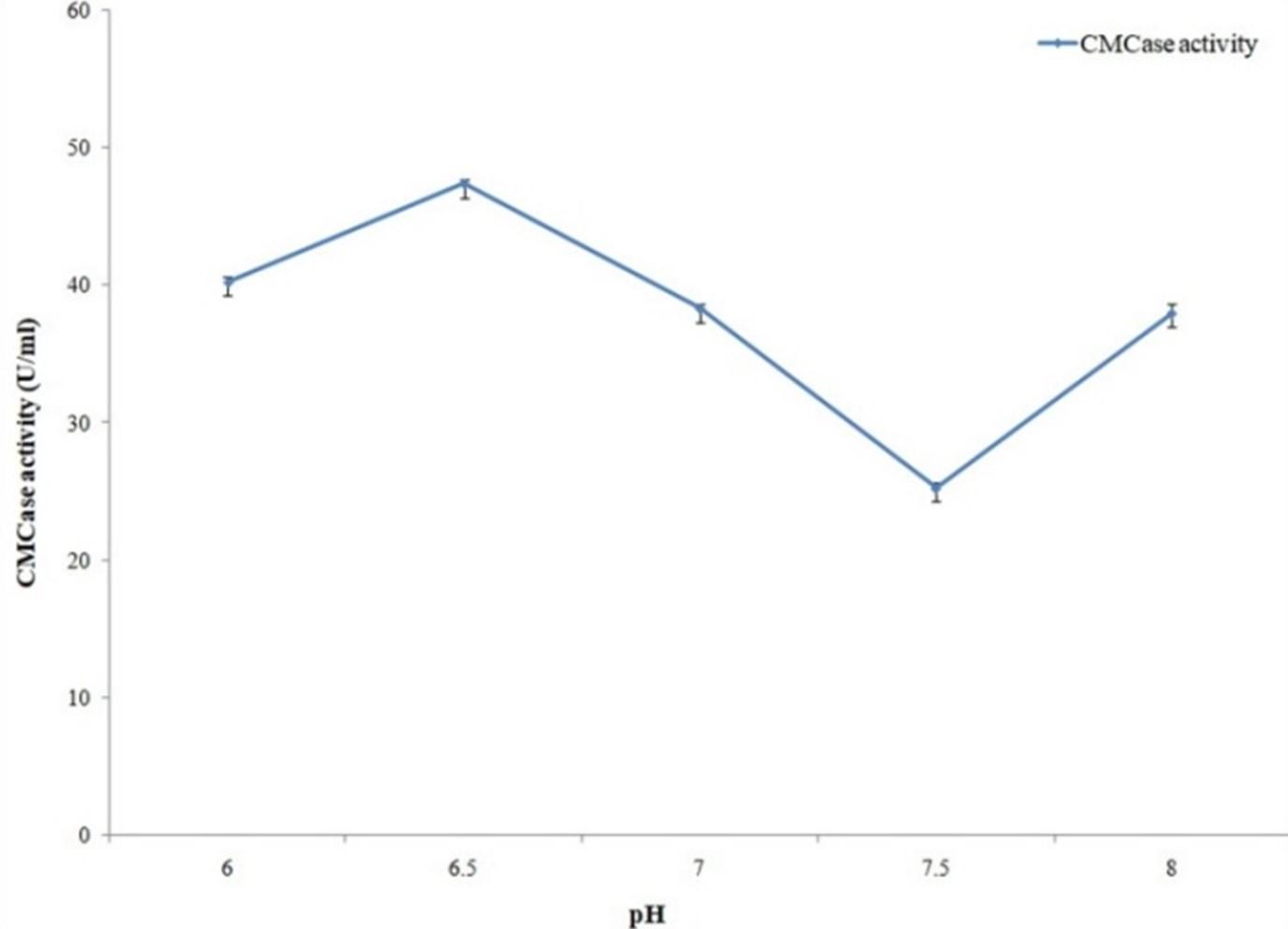
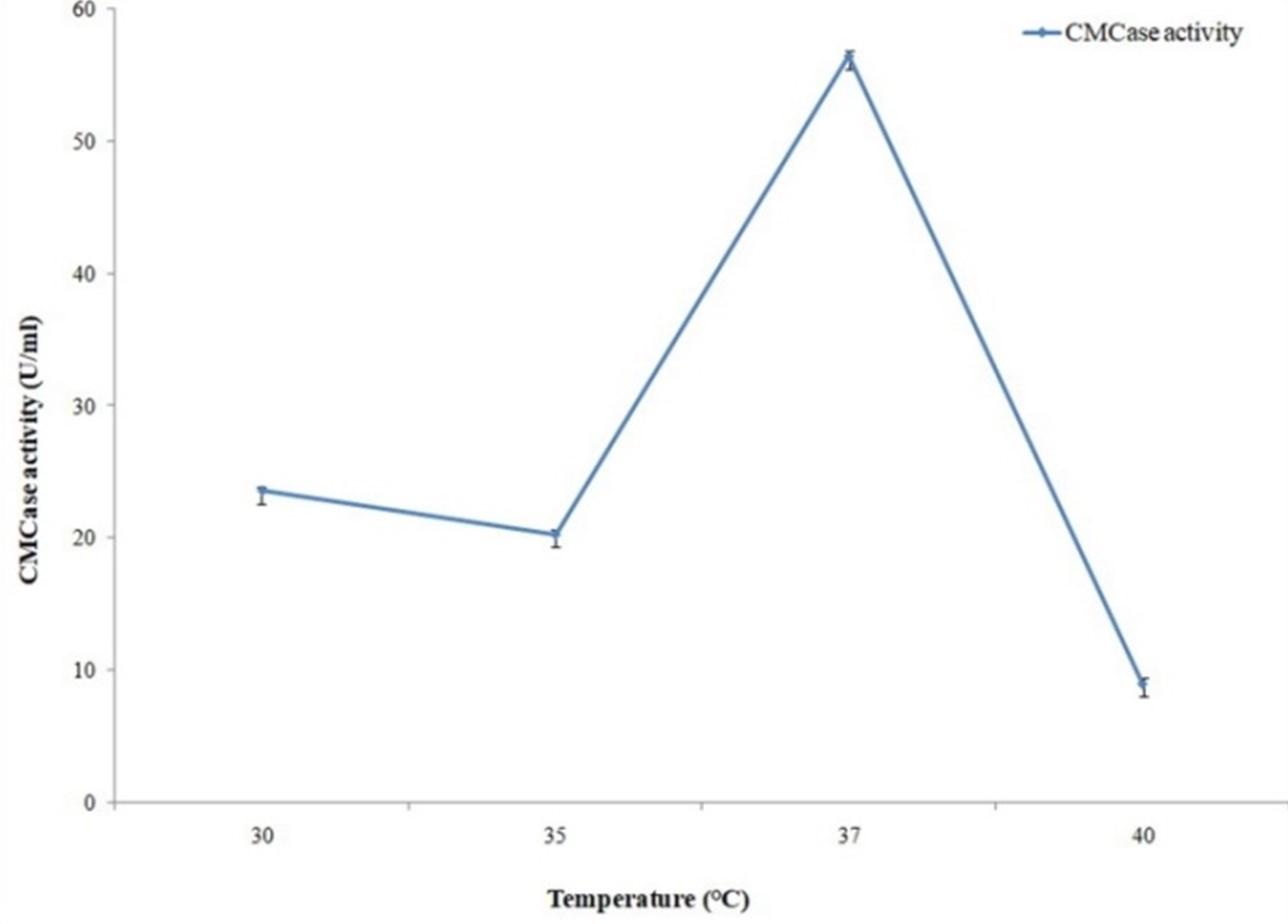
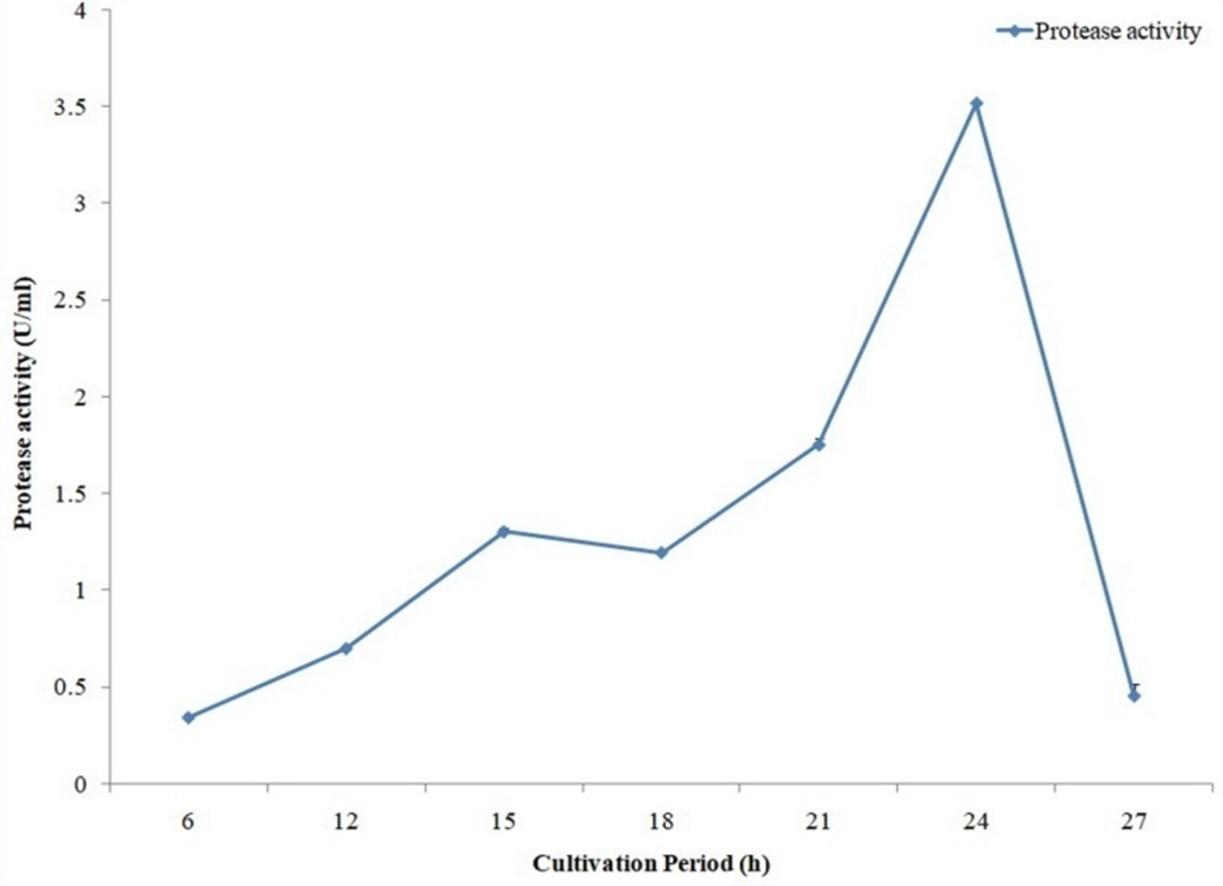
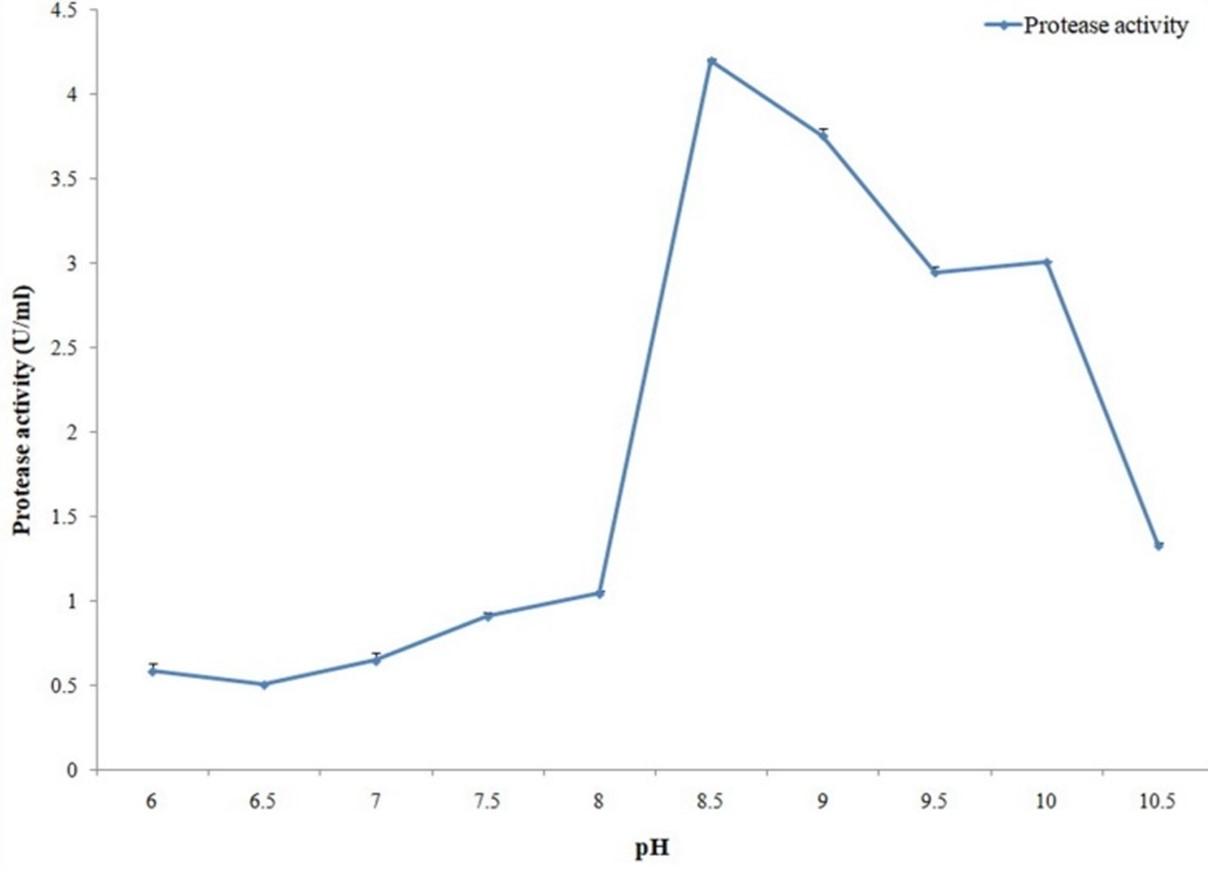
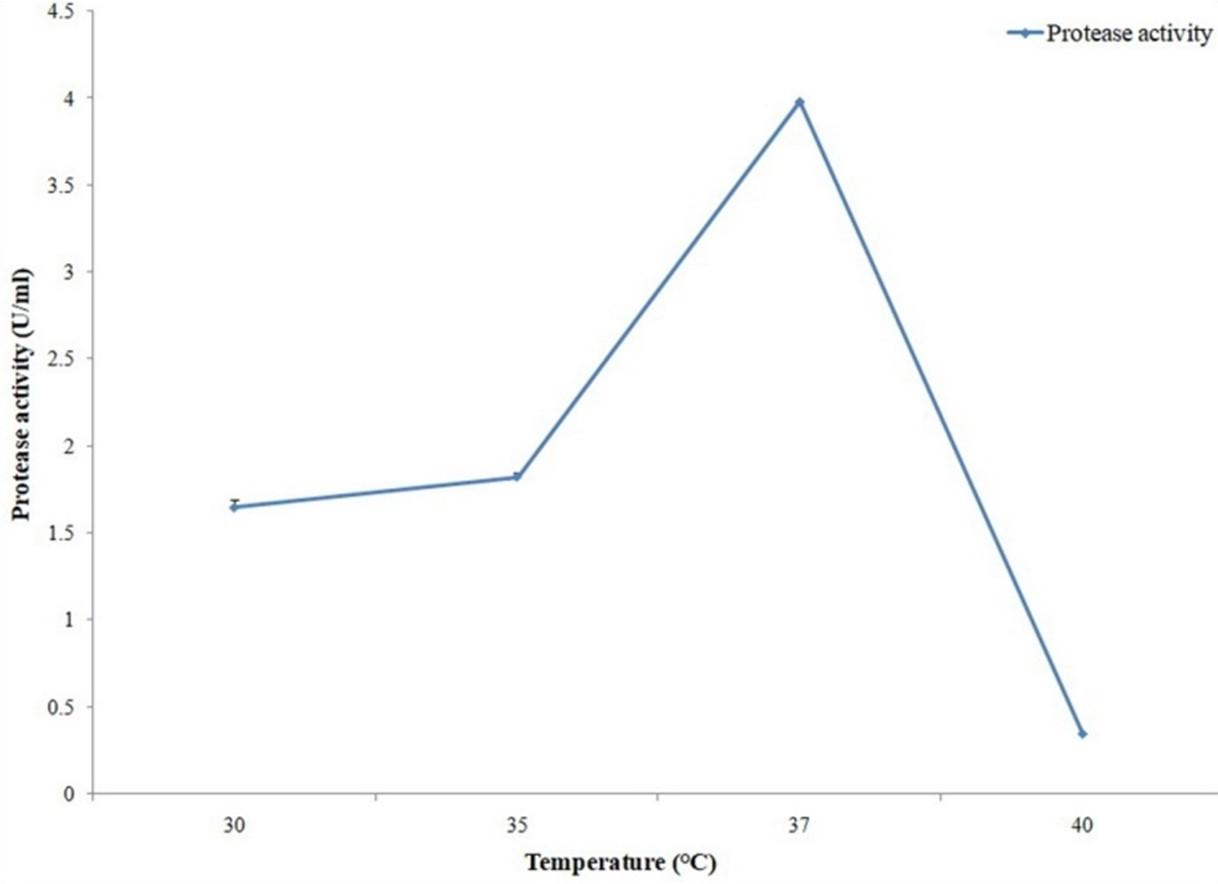
Optimization of cellulase and protease assay condition
The effect of pH on cellulase and protease activities was evaluated by carrying out both activity assays in different pH conditions that were adjusted by using different buffers. Maximum cellulase activity was observed with pH 6.5 (Figure 11) while in case of protease it was pH 6.
Cellulase and protease activity assays were conducted in different reaction temperatures where maximum activities were observed with 60°C for cellulase (Figure 12) and with 70°C for protease (Figure 13).
Cellulase and protease activities in presence of different metal ions were tested by using 5 important and common metal ions. Maximum cellulase activity was observed with Ca2+ (Figure 14) and maximum protease activity was observed with Cu2+ (Figure 15). In this study, Analysis of variance (ANOVA) was performed using SPSS Version 25 to investigate the significance of the treatment effect means. In addition, multiple comparison tests were also conducted to explore the pairwise comparison between or among treatment means. For multiple comparisons test most significant difference (MSD) was calculated and made pairwise comparison at t 0.01 with error degrees of freedom.
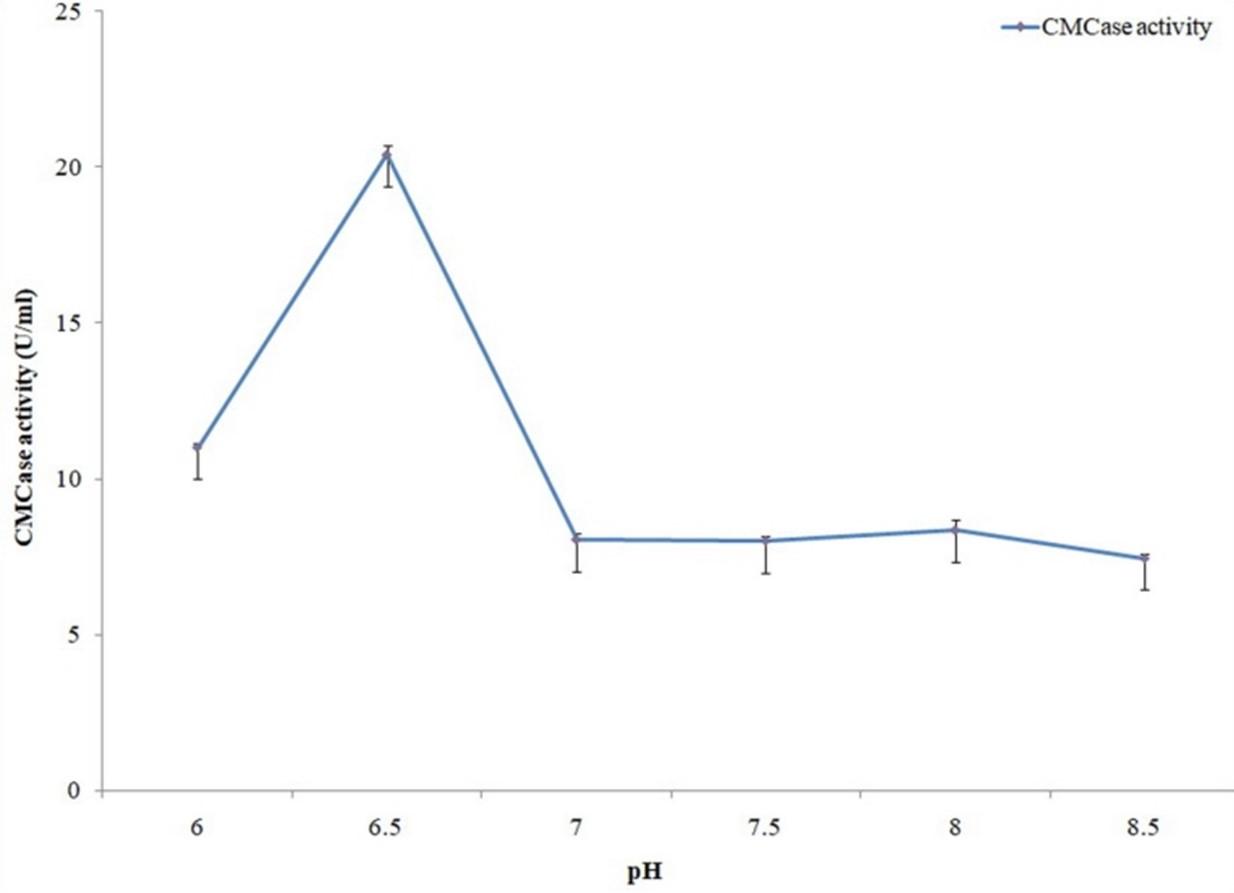
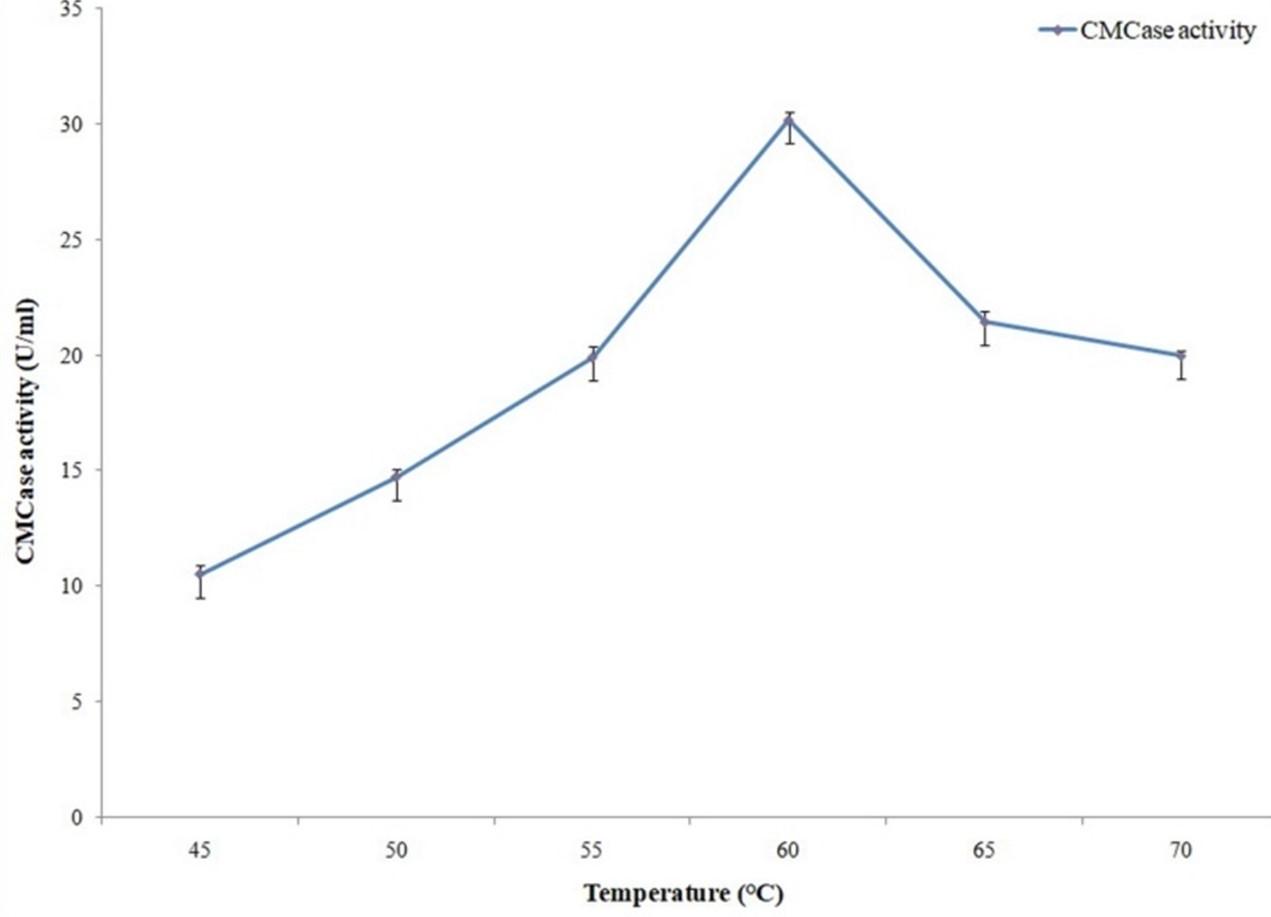
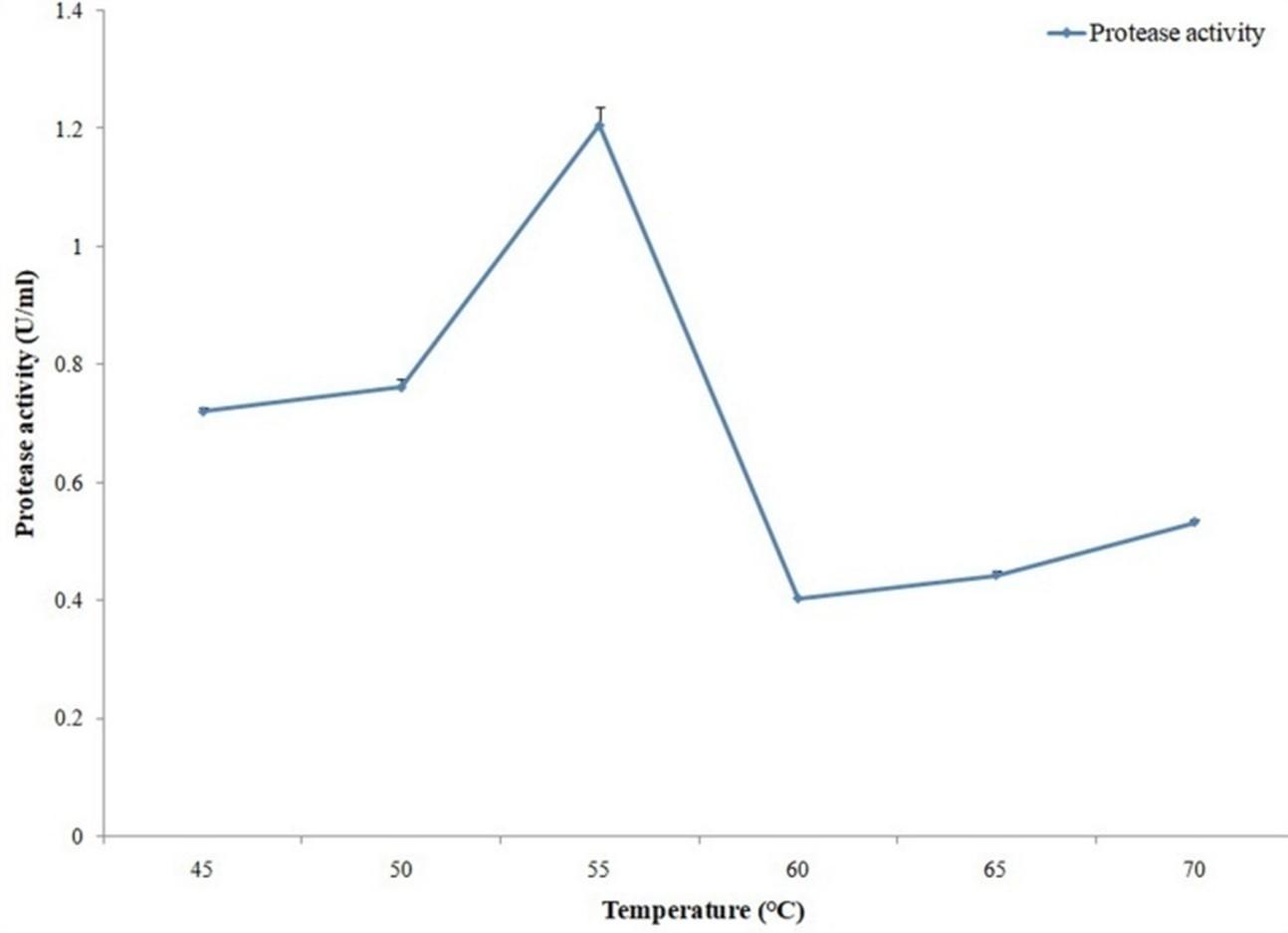
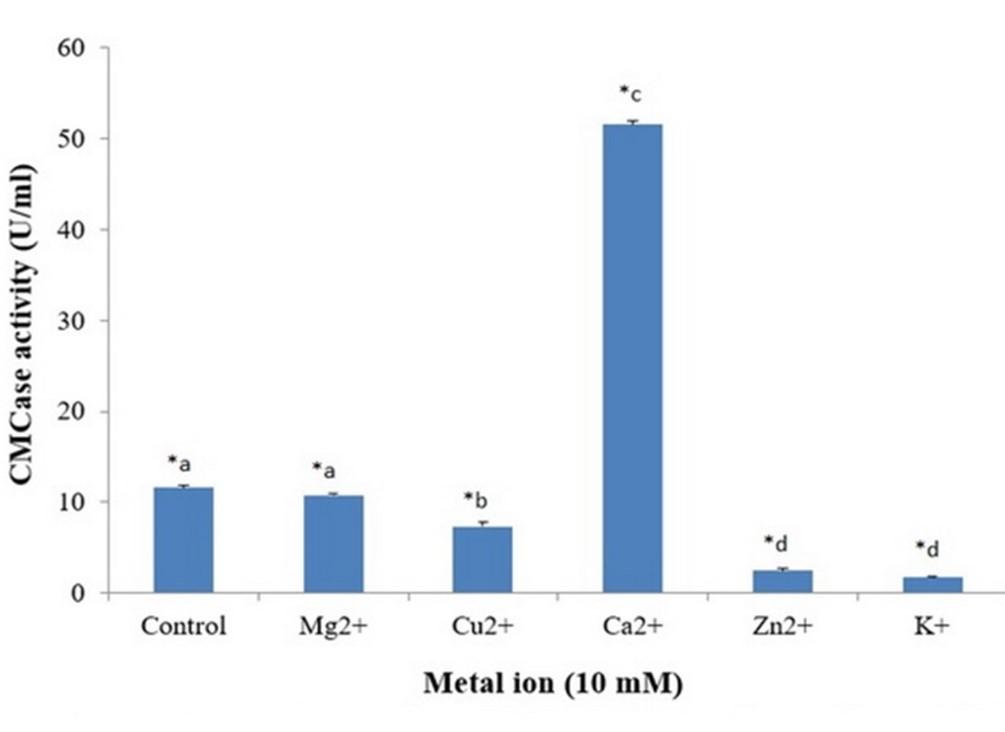
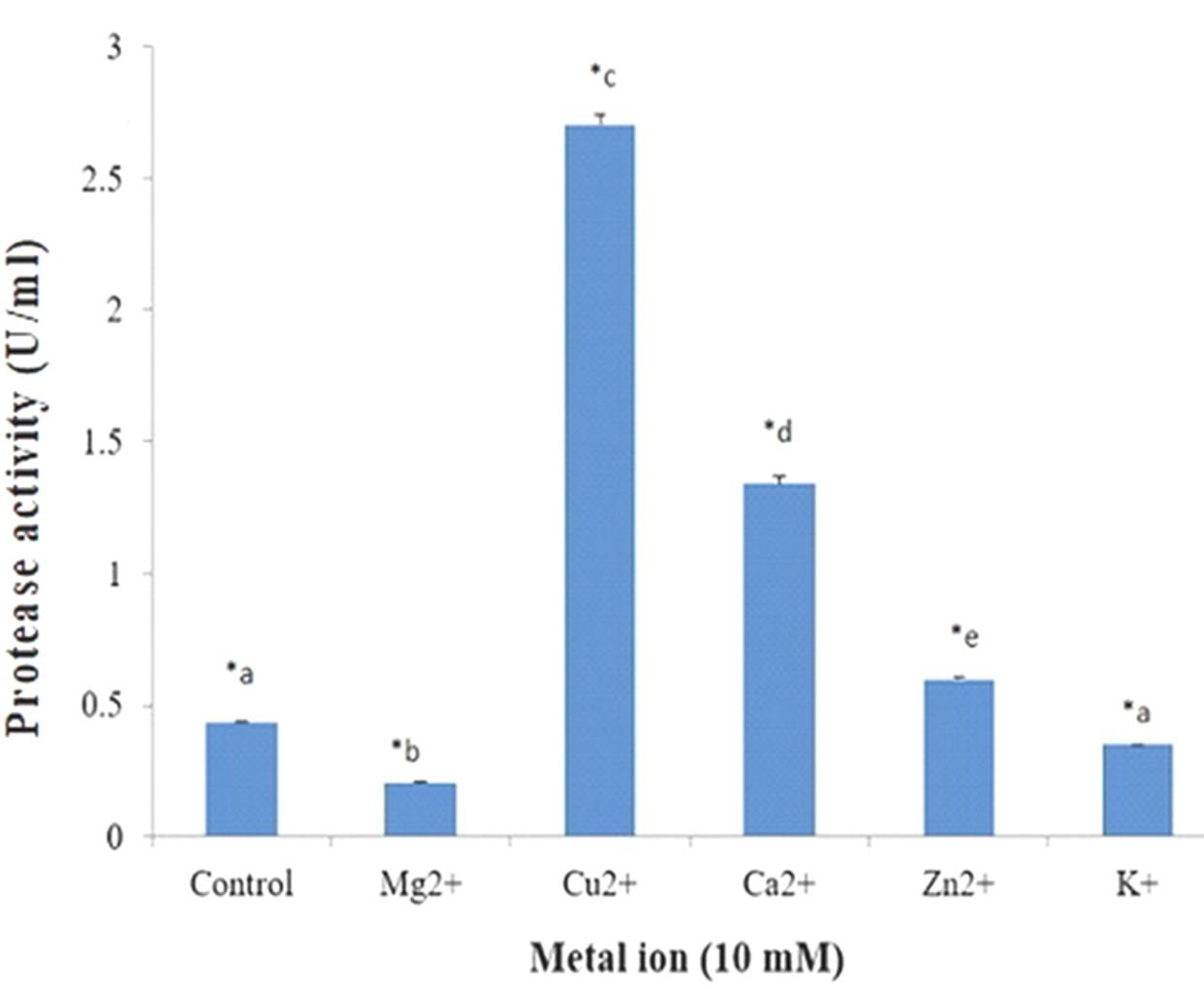
DISCUSSION
Microorganisms have been regarded as treasure sources of useful enzymes. They are usually capable of digesting insoluble nutrient materials such as cellulose, protein and starch [14]. Different microorganisms have been reported to produce cellulase but bacteria which have high growth rate as compared to fungi have good potential to be used in cellulase production [15]. In recent years, there is renewed interest in the study of proteolytic enzymes, mainly due to the recognition that these enzymes not only play an important role in the cellular metabolic processes but have also gained considerable attention in the industrial community such as in detergents, leather industry, food industry, pharmaceutical industry, and bioremediation processes [16, 7].
In the present study, an attempt was made to screen cellulolytic and proteolytic bacteria from 260 bacterial isolates that were isolated from different locations of Sylhet, Bangladesh. Among 260 different colonies, 6 were identified as potential cellulolytic bacteria based on the halo zone formed by the isolates in CMC agar media. Proteolytic activities of the 260 bacterial isolates were also tested using skim milk agar and 15 bacterial isolates were identified as potential proteolytic bacteria. Interestingly, among the identified cellulolytic and proteolytic bacteria 6 were capable of degrading both cellulose and protein. In a previous research work, Ariole et al. (2014) identified 4 bacteria that had both cellulolytic and proteolytic activity [17]. Among the 6 selected isolates, strain S32 was found to be the best for degrading both cellulose and protein.
S32 appeared as Gram negative, methyl red positive, Voges–Proskauer negative, catalase test negative, sugar fermentation test positive, indole test positive and citrate test negative. The biochemical tests results indicate that S32 bacterial strain is a species of the genus Aeromonas [18, 19].
Molecular identification of the selected bacterial isolate was also done. 16S rDNA sequence analysis revealed maximum similarity with Aeromonas hydrophila. Bacterial isolate S32 is a strain of Aeromonas hydrophila and was named as Aeromonas hydrophila ASM-S32.
Optimization of culture conditions is very important for maximum microbial growth and enzyme production by microorganisms [20]. Among the physical and chemical parameters, optimum temperature, pH ranges are the most important for enzyme production by microbes [21, 22]. 18 hours cultivation period resulted in maximum cellulase production for A. hydrophila ASM-S32. To identify optimum pH for cellulase production, different pH (6 to 8.5) conditions were applied on cellulase production media and best result was obtained with pH 6.5. Another important parameter for enzyme production is temperature. Maximum cellulase production resulted from the production media kept at 37° C temperature. 4-fold higher cellulase production was found when optimized conditions were used. A research work conducted in Nigeria reported that isolates exhibited optimal cellulolytic activities at 32.6 ± 6.2°C and pH 6.29 ± 0.9 [23]. Ariffin et al. (2008) reported optimal cellulase activity of Bacillus pumulus EB3 at 37°C and pH 7 which is also similar to the present study [24].
Activity of the cellulase enzyme was measured with different pH (6 to 8.5), temperatures (45°, 50°, 55°, 60°, 65°, 70°C) and metal ions in the present study. Optimum cellulase activity was obtained with pH 6.5 and 60°C temperature. Almost similar results were obtained by Liang et al. (2010) who reported optimum cellulase activity for Anoxybacillus sp. 527 with pH 6.0 and 70°C temperature [25]. Since thermostability of an enzyme is an important parameter for industrial utilization [26], highest cellulolytic activity at 60°C temperature from our isolate can become industrially more demandable in the near future.
Metal ions also play an important role for cellulase activity. Liang et al. (2010) found maximum cellulase activity from Anoxybacillus sp. 527 in presence of Ca2+ ion [25]. Similar results have been found with A. hydrophila ASM-S32 where Ca2+ ion yielded maximum cellulase activity.
To obtain the optimum conditions for protease production by A. hydrophila ASM-S32, experiments were also carried out for different cultivation periods, temperatures and pH. Among different cultivation periods, maximum protease production was found with 24 hours incubation. Protease production by A. hydrophila ASM-S32 was carried out with different pH and the best result was obtained with pH 8.5. Maximum protease production resulted from 37°C temperature. Protease production was increased by 1.2-fold at optimized conditions as compared to unoptimized conditions. Koka and Weimer, (2000) reported metalloprotease production from Pseudomonas fluorescens RO98 with an optimum pH 5.0 and incubation temperature of 35ºC [27]. Singh et al. (2015) found that the optimum temperature for protease production from Bacillus strains BP1 and BP2 was 37±2°C [28].
Optimum activity of protease enzyme was measured with different pH (6 to 10.5), temperatures (45, 50, 55, 60, 65, 70 and 75°C) and metal ions in this study. Maximum protease activity was obtained with pH 6.0 and 70°C temperature. In the case of metal ions, optimum protease activity was obtained with Cu2+ ion. In a recent research maximum protease activity was obtained from Aspergillus oryzae with pH range 5.0–5.5 and temperature range for optimum activity was 55–60°C [29]. Proteases with activity and stability at high temperature are attention-grabbing for bioengineering and biotechnological applications [30]. In the present study, protease produced from the strain A. hydrophila ASM-S32 shows maximum proteolytic activity at 70°C temperature which can make it industrially more preferable as thermostable proteolytic enzyme.
CONCLUSIONS
Among the identified 6 cellulolytic and 15 proteolytic bacteria strains, A. hydrophila ASM-S32 was found to be the most potential one in producing extracellular cellulase and protease enzymes. A. hydrophila ASM-S32 produced maximum cellulase and protease at 37°C after 18 and 24 hours cultivation period with an initial pH of 6.5 and 8.5 respectively. Optimum cellulase and protease activity was observed in presence of Ca2+ and Cu2+ ions respectively. Interestingly, both cellulase and protease enzymes produced by A. hydrophila ASM-S32 exhibited activity at a very high temperature of 60°C and 70°C respectively. Therefore, it can be concluded that A. hydrophila ASM-S32 can be a potential producer of extracellular cellulase and protease and can be highly demandable from industrial point of view.
ACKNOWLEDGEMENT
The Authors are grateful to the Department of Genetic Engineering and Biotechnology, Shahjalal University of Science and Technology, Bangladesh for the laboratory facilities towards completing the study.
AUTHOR CONTRIBUTIONS
SMAS planned the work and analyzed the experimental data that led to the manuscript; SC, ZFJ and AH produced and analyzed the experimental data; SA, SC, ZFJ, AI, PKS and SMAS participated in the interpretation of the results; SMAS, SA, SC and ZFJ wrote the paper. All authors read and approved the final manuscript.
CONFLICTS OF INTEREST
The authors declare that no conflict of interest exists.
References
- [1]Kirk O, Borchert TV, Fuglsang CC. Industrial enzyme applications. Curr opin biotechnol. 2002; 13(4):345-351.
- [2]Kumar P, Sharma SM. Enzymes in green chemistry: The need for environment and sustainability. Int J Appl Res. 2016; 2:337-341.
- [3]Rao MB, Tanksale AM, Ghatge MS, Deshpande VV. Molecular and biotechnological aspects of microbial proteases. Microbiol Mol Biol Rev. 1998; 62(3):597-635.
- [4]Singh A. Engineering enzyme properties. Indian J Microbiol. 1999; 39(2):65-77.
- [5]Singh A, Kuhad RC, Ward OP. Industrial application of microbial cellulases. Lignocellulose Biotechnology: Future Prospects. 2007; 345-58.
- [6]Saranraj P, Stella D, Reetha D. Microbial cellulases and its applictions. Int J Biochem Biotechnol. Sci. 2012; 1:1-2.
- [7]Gupta R, Beg Q, Lorenz P. Bacterial alkaline proteases: molecular approaches and industrial applications. Appl Microbiol Biotechnol. 2002; 59(1):15-32.
- [8]Anwar A, Saleemuddin M. Alkaline‐pH‐acting digestive enzymes of the polyphagous insect pest Spilosoma obliqua: stability and potential as detergent additives. Biotechnol Appl Biochem. 1997; 25(1):43-6.
- [9]Oskouie SF, Tabandeh F, Yakhchali B, Eftekhar F. Enhancement of alkaline protease production by Bacillus clausii using Taguchi experimental design. Afr J Biotechnol. 2007; 6(22).
- [10]Kasana RC, Salwan R, Dhar H, Dutt S, Gulati A. A rapid and easy method for the detection of microbial cellulases on agar plates using Gram’s iodine. Curr Microbiol. 2008; 57(5):503-7.
- [11]Maki ML, Broere M, Leung KT, Qin W. Characterization of some efficient cellulase producing bacteria isolated from paper mill sludges and organic fertilizers. Int J Biochem Mol Biol. 2011; 2(2):146.
- [12]Bhunia B, Dutta D, Chaudhuri S. Selection of suitable carbon, nitrogen and sulphate source for the production of alkaline protease by Bacillus licheniformis NCIM-2042. Not Sci Biol. 2010; 2(2):56-9.
- [13]Folin O, Ciocalteu V. On tyrosine and tryptophane determinations in proteins. J Biol Chem. 1927; 73(2):627-50.
- [14]Ann Suji H, Palavesam TA, Immanuel G, & Suthin Raj. Production of different enzymes by gut microflora. Int J Sci Nat. 2014; 5(1), pp.28-32.
- [15]Immanuel G, Dhanusha R, Prema P, Palavesam A. Effect of different growth parameters on endoglucanase enzyme activity by bacteria isolated from coir retting effluents of estuarine environment. Int J Environ Sci Technol. 2006; 3(1):25-34.
- [16]Anwar A. and Saleemuddin M. Alkaline proteases: a review. Bioresour technol. 1998; 64(3), pp.175-83.
- [17]Ariole CN, Nwogu HA, Chuku PW. Enzymatic activities of intestinal bacteria isolated from farmed Clarias gariepinus. Intel J Aquacult. 2014; 4.
- [18]Von Graevenitz A. Practical substitution for the indole, methyl red, Voges-Proskauer, citrate system. J Appl Microbiol. 1971; 21(6):1107-9.
- [19]Erdem B, KARİPTAŞ E, Cil E, IŞIK K. Biochemical identification and numerical taxonomy of Aeromonas isolated from food samples in Turkey. Turkish J Biol. 2011; 35(4):463-72.
- [20]Kathiresan K, Manivannan S. Amylase production by Penicillium fellutanum isolated from mangrove rhizosphere soil. Afr J Biotechnol. 2006; 5(10).
- [21]Bose K, Das D. Thermostable alpha-amylase production using Bacillus licheniformis NRRL B14368. Indian J Exp Biol. 1996; 34(12):1279-82.
- [22]Gupta R, Gigras P, Mohapatra H, Goswami VK, Chauhan B. Microbial α-amylases: a biotechnological perspective. Process Biochem. 2003; 38(11):1599-616.
- [23]Otajevwo FD, Aluyi HS. Cultural conditions necessary for optimal cellulase yield by cellulolytic bacterial organisms as they relate to residual sugars released in broth medium. Mod Appl Sci. 2011; 5(3):141.
- [24]Ariffin H, Hassan MA, Shah UK, Abdullah N, Ghazali FM, Shirai Y. Production of bacterial endoglucanase from pretreated oil palm empty fruit bunch by Bacillus pumilus J Biosci Bioeng. 2008; 106(3):231-6.
- [25]Liang Y, Feng Z, Yesuf J, Blackburn JW. Optimization of growth medium and enzyme assay conditions for crude cellulases produced by a novel thermophilic and cellulolytic bacterium, Anoxybacillus 527. Appl Biochem Biotechnol. 2010; 160(6):1841-52.
- [26]Momeni MH, Goedegebuur F, Hansson H, Karkehabadi S, Askarieh G, Mitchinson C, Larenas EA, Ståhlberg J, Sandgren M. Expression, crystal structure and cellulase activity of the thermostable cellobiohydrolase Cel7A from the fungus Humicola grisea var. thermoidea. Acta Cryst. D. 2014; 70(9):2356-66.
- [27]Koka R, and Weimer BC. Isolation and characterization of a protease from Pseudomonas fluorescens J Appl Microbiol. 2000. 89(2), pp.280-8.
- [28]Singh P, Rani A, Chaudhary N. Isolation and characterization of protease producing Bacillus from soil. Int J Pharm Sci Res. 2015; 6(4), pp.633-9.
- [29]de Castro RJ, Sato HH. Protease from Aspergillus oryzae: biochemical characterization and application as a potential biocatalyst for production of protein hydrolysates with antioxidant activities. J Food Process. 2014; 2014.
- [30]Sellami-Kamoun A, Haddar A, Ali NE, Ghorbel-Frikha B, Kanoun S, Nasri M. Stability of thermostable alkaline protease from Bacillus licheniformis RP1 in commercial solid laundry detergent formulations. Microbiol Res. 2008; 163(3):299-306.