Coordination of elicitors and ascorbate-glutathione cycle: A vital nexus for mitigating post-harvest injury and losses of cultivated fruits
Abstract
Fruit losses and waste are predominantly caused by postharvest stresses, with their management traditionally reliant on synthetic compounds. The extensive utilization of these compounds can cause harmful impacts on both human health and the environment, necessitating a reduction in their use. Recent advancements have led to the development of novel, benign strategies using natural or biologically derived compounds. These compounds, known as 'elicitors,' have the strength to instigate the plant's defense mechanism, enhancing the fruit’s resilience counter to future stress and reducing postharvest spoilage. In recent years, the impact of the plant defense system concerning elicitor compounds has been measurable through physiological parameters or the enzymatic activity of molecular markers. Antioxidant activity, a crucial parameter to determine fruit quality, shelf-life, and tolerance to biotic and abiotic stresses, significantly functions in stress response and maintaining postharvest quality. Elicitors can prompt endurance in fruits towards diverse environmental stresses by promoting the biosynthesis of antioxidant components and enhancing antioxidant capability through the ascorbate-glutathione (AsA-GSH) cycle. Recent findings have exhibited that applying chemical elicitors can enhance antioxidant activity by augmenting the content of AsA and GSH to regulate redox stability, thereby maintaining quality in harvested fruits. This review summarises recent findings demonstrating how elicitor spray can enrich antioxidant activity in harvested fruits by accelerating the synthesis of antioxidants by activating other defense mechanisms providing insight into fruit defense mechanisms induced under various elicitors and their prospects in postharvest biology.
INTRODUCTION
Fruits are abundant in beneficial compounds, playing a pivotal role in a nutritious diet for the overall well-being of human consumption. They are often harvested when green mature to minimize postharvest losses from texture softening, desiccation, and pathogen infection. However, research shows that fruits ripened naturally have better quality, especially in aroma, than those ripened postharvest [1, 2]. Fruits are highly perishable, and various factors can impact their original attributes. Global fruit post-harvest losses are estimated between 25 and 50% of total production. Even under ideal storage conditions, they are susceptible to decomposing during the post-harvest period [3]. A recent FAO report highlighted that fruits have the second highest value of losses and waste (~22%) in the food supply chain, after roots, tubers, and oil-bearing crops, due to their perishable nature [4]. Metabolism of Postharvest fruits is influenced by endogenous (intrinsic physiology) and exogenous factors (abiotic and biotic). Ripening and senescence can vary based on genotype through interaction with these factors [5, 6]. In response, inorganic chemical substances have been traditionally used to manage these stresses during the postharvest period. Their frequent use has led to the development of microbial resistance and pesticide residues in fruits, which poses health risks raising environmental concerns.
Several government policies along with the Food and Agriculture Organization (FAO) recommended limiting the use of these inorganic harmful pesticides in crops. Research is ongoing to identify cost-effective, safe, environmentally friendly alternatives for controlling fruit diseases. Elicitors or resistance signaling inducers have been a focus of scientific research for over two decades as potential replacements for pesticides [7, 8].
Fruits can experience oxidative stress as they ripen and undergo senescence after harvest, which results in higher production of ROS, which are natural by-products of metabolism, growth and development. They are distributed across various organelles within the fruit cells. Plants have developed nonenzymatic and enzymatic mechanisms to regulate the balance between ROS generation and scavenging under normal physiological conditions. However, specific abiotic stressors can produce excessive ROS during oxidative stress, resulting in collateral destruction of cell structures, membrane lipid peroxidation, and impairment of proteins and DNA, which results in abnormal fruit ripening. Early responses in postharvest fruit are often due to disruptions in the system that produces and scavenges ROS [9]. Antioxidants like ascorbate (AsA) and glutathione (GSH) play a key role in combating ROS, along with superoxide dismutase (SOD), peroxidase (POD), catalase (CAT), and ascorbate peroxidase (APX). The AsA-GSH cycle is crucial for ROS removal during postharvest storage. Fruits with sufficient levels of GSH, AsA, APX, GR, MDHAR, and DHAR involved in the AsA-GSH pathway exhibit improved tolerance to oxidative stress. Numerous investigations have focused on the importance of the AsA-GSH cycle in balancing the postharvest standard of fruits and holding up their senescence with elicitors (physical/chemical) application like plant hormones, nanoparticles, compounds gas, ray, etc., which also can enhance the ROS scavenging power, reducing ROS amount in host fruits to mitigate fruit damage and coordinate the induction of other defense response regulatory pathways [1, 7, 8, 10-17]. This review highlights recent advancements in using different elicitors on fruits after harvest to enhance the stress-reducing AsA-GSH cycle. Additionally, it explores how these elicitors activate diversified mechanisms with relevant molecular pathways in fruits, providing insights into the broader context of plant defense networks.
POST-HARVEST STRESS IN FRUITS: ROS, A KEY FACTOR
Regular consumption of fresh fruits provides essential nutrients and antioxidants that help reduce the risks of lifestyle-related diseases. However, after harvesting fruits and vegetables, their storage duration, and shelf-life are important for the agriculture industry. Managing postharvest fruits involves balancing ROS and antioxidants, which can be challenging due to the plant’s response to stress signals. Excessive ROS levels can damage cells and proteins, leading to lipid peroxidation. Maintaining a sustainable balance between ROS and antioxidants is important to prevent stress in postharvest produce. Signalling chemicals alternatively known as elicitors can help increase antioxidant production and protect against postharvest handling and distribution. This can be achieved by upregulating the Ascorbate-Glutathione (AsA-GSH) cycle [7, 8, 18].
ROLE OF AsA-GSH CYCLE IN MITIGATING ROS-MEDIATED STRESS
ROS is produced during aerobic reactions when oxygen is partially reduced or activated. ROS are a group of reactive components that include “superoxide (O2−), hydroxyl (OH•), and peroxyl (ROO•), as well as nonradical forms like hydrogen peroxide (H2O2), singlet oxygen (1O2), and ozone (O3). ROS play crucial dual functions in plants: at moderate levels, they work as signaling molecules to induce responses to abiotic stress for adaptation, while excessive amounts can cause oxidative bursts. At the highest point, this oxidative damage can lead to lipid peroxidation of membranes and protein and nucleic acid degradation, potentially culminating in the oxidative destruction of the cell. ROS are produced in several cellular locations, including the chloroplast, mitochondrion, cell membranes, and apoplast. Upon initial stress perception, plants synthesize specific hormones (salicylic acid—SA, jasmonic acid—JA, ethylene—ET, and abscisic acid—ABA) tailored to the required physiological response. These hormones propagate through the plant system, initiating signaling pathways that modulate gene expression changes. Consequently, antioxidant enzymes are synthesized to scavenge the elevated ROS levels induced by oxidative stress, thus supporting redox potential and plant survival under stress conditions. The cross-talk among these components improves signaling relations and stabilizes the plant defense system. [19]. Plant’s antioxidants in organelles neutralize the ROS. Excessive amounts of ROS production can cause oxidative damage [20, 21].
BIOSYNTHESIS OF ASCORBATE AND GLUTATHIONE
AsA biosynthesis, one of the most ancient biological pathways, is crucial for various primitive life processes on Earth. In plants, AsA is mainly produced via the L-galactose pathway, also referred to as the Smirnoff–Wheeler pathway. This pathway begins with converting D-glucose-6-phosphate to D-fructose-6-phosphate, followed by a sequence of enzymatic reactions that culminate in the synthesis of AsA. The pathway's key enzymes include phosphoglucose isomerase (PGI), phosphomannose isomerase (PMI), and GDP-mannose pyrophosphorylase (GMP), among others. Notably, the enzyme L-galactono-1,4-lactone dehydrogenase (GLDH), crucial in the final step, is located in the mitochondrial inner membrane, indicating mitochondrial involvement in AsA synthesis. While the L-galactose pathway is predominant, alternative pathways such as the L-gulose and D-galacturonic acid pathways also contribute to AsA production, underscoring the complexity and versatility of AsA biosynthesis linked to cell wall formation [20, 21].
Glutathione (GSH), a ubiquitous tripeptide composed of glutamine, cysteine, and glycine, is a potent antioxidant and a vital metabolite with diverse roles in plants, ranging from antioxidant defense to cell cycle regulation. GSH synthesis occurs in two ATP-dependent enzymatic steps, starting with γ-glutamylcysteine formation, followed by its conversion to GSH, facilitated by GSH synthetase. GSH is crucial for cellular antioxidant capacity, particularly under abiotic stress, and AsA regeneration in the AsA-GSH cycle. This cycle is integral for maintaining cellular redox homeostasis and enabling plants to withstand various stress conditions [20-22].
ENZYMES OF AsA-GSH INVOLVED IN REDOX SIGNALISING
AsA/DHA and GSH/GSSG play vital roles as redox managers in maintaining the redox balance within plant cells. Ascorbate is key in scavenging ROS and cellular processes such as “electron donation to photosystem II (PSII), violaxanthin de-epoxidation, and α-tocopherol reductive quenching”. The AsA pool directly reduced MDHA by ferredoxin at photosystem I (PSI), Monodehydroascorbate reductase (MDHAR), and dehydroascorbate (DHA) reduction via GSH-dependent dehydroascorbate reductase (DHAR) activity. The redox potential of GSH is influenced by its concentration and GSH/GSSG ratio. Both AsA and GSH are essential components of the cellular redox homeostasis network. In this cycle, AsA donates electrons to ascorbate peroxidase (APX), which detoxifies H2O2 by its high affinity for H2O2, allows it to scavenge ROS effectively even at low concentrations, producing DHA, which is then recycled to maintain a high AsA/DHA ratio. If DHA is not reduced, it may be irreversibly hydrolyzed, diminishing the AsA redox pool’s efficacy. Ascorbate oxidase (AO) creates a redox gradient across the plasma membrane and facilitates apoplastic and cytoplasmic signalling. Conversely, the GSH/GSSG couple balances the intracellular redox potential and signalling. Thus, understanding the distribution of GSH across different cellular organelles is crucial for effective signalling, cellular metabolism, and fruit defense.
SOD catalyses the dismutation of the superoxide anion (•O2−) to form H2O2 and O2 to counteract oxidative stress [31]. SOD works in close synchrony with additional enzymes, for instance, catalase (CAT) and plant peroxidases (POX), to prevent the formation of more harmful ROS through the Haber-Weiss reaction. At low concentrations of H2O2, this enzyme stays inactive. However, when H2O2 concentrations increase, a second molecule of H2O2 functions as a reductant for the enzyme producing water and oxygen as outputs. Compared to ascorbate APX, CAT has a higher Km for H2O2, making it more active at higher concentrations. APX is located in multiple subcellular compartments, allowing finely tuned scavenging activity. POXs, or class III peroxidases, function in the extracellular space to scavenge H2O2. Regarding antioxidant mechanisms, the interaction of ascorbate-glutathione molecules maintains redox balance through the Foyer-Halliwell-Asada also known as AsA-GSH pathway. Flavonoids, phenolic compounds, and POX are backup defense against excessive H2O2 [19, 20].
GPX catalyzes the conversion of H2O2 to water and lipid alcohols, respectively. It has been considered as a biomarker for intracellular oxidative stress. Glutathione reductase (GR), a flavoprotein oxidoreductase NAD(P)H-dependent enzyme, is crucial in the AsA pathway, reducing GSSG to reduced GSH. Ascorbate peroxidases (APX), a class I heme peroxidase familiar as ascorbate-dependent peroxidase, scavenges H2O2 and senses redox alterations within plant cells, playing an essential role in the “Foyer-Halliwell-Asada pathway”. It uses ascorbate to convert H2O2 to water, producing mono dehydroascorbate reductase (MDHA) as an intermediate. MDHA, which is unstable and quickly converted to DHA, is reduced and recapitulates ascorbate by MDHAR using NADH or NADPH. This enzyme's activity, reported in diverse cellular compartments, namely chloroplasts and mitochondria, is vital for maintaining a reduced ascorbate pool. DHAR breaks DHA to ascorbic acid, utilizing reduced GSH, which is critical for maintaining sufficient ascorbate levels. The continuous recycling of small soluble antioxidants like ascorbate and GSH is crucial for maintaining cellular redox balance. The antioxidant defense system's protective role extends to safeguarding unsaturated membrane lipids, nucleic acids, enzymes, and other cellular structures, making it a topic of significant interest in plant growth and development research [19, 20].
From a recent study, GSH (5 mM) significantly mitigated weight loss in mangoes by acting as an antioxidant. When applied as an edible coating on the fruit peel, it maintains low total soluble solids (TSS), inhibiting the rapid increase in sugar content during ripening towards lower lipid peroxidation indicated by lower malondialdehyde (MDA) levels maintaining a high GSH level and favourable GSH/GSSG ratio help to regulate redox balance to oxidative stress homeostasis during ripening, and post-harvesting and storage of fruits. The impact of exogenous GSH on chilling injury (CI) in postharvest bell pepper fruits stored at low temperatures was mitigated by its role in stabilizing membranes and maintaining organelle integrity through the AsA-GSH cycle. Cold stress is mitigated by enhancing the expression of Asa-GSH cycle-related genes CaGR2, CaAPX1, CaGR2, CaMDHAR1 and CaDHAR1.
ELICITORS AND AsA-GSH CYCLE FOR MITIGATING POSTHARVEST INJURY AND DAMAGES
Oxidative burst (ROS) is a common response to stress in fruits, leading to the rapid production of ROS. Induced resistance is a new approach to managing postharvest stress by activating fruit immune responses with external elicitors. This process involves signal transduction to enhance stress resistance genes and biosynthesis of secondary metabolites. Elicitors can influence the antioxidant defense system by upregulating the AsA-GSH cycle c0-ordinated other defense pathways (Figure 1) (Table 1) [23, 24].
Chitosan
Chitosan is a natural biopolymer derived from crustaceans, insect cuticles, and fungal cell walls consisting of N-acetyl-D-glucosamine and D-glucosamine connected by β-1,4-glycosidic bonds well-known for its biocompatibility, biodegradability, antibacterial properties. Recent studies have highlighted chitosan, an elicitor of plant defense, enhancing plant resistance to multiple stresses by activating defense-related responses accumulating the antioxidants through synthesis (Phenolics, flavonoids), and upregulating defense enzymes like polyphenol oxidase (PPO), phenylalanine ammonia-lyase (PAL), nonenzymatic components, strengthening plant cell walls, providing a mechanical barrier to biotic stresses like pathogen attacks, boosting the effectiveness of defense enzymes like β-1,3-glucanase, chitinase, and chitosanase named pathogenesis-related (PR) proteins, enhance plant resistance against different pathogens by breaking down fungal wall polysaccharides and attacking invading pathogens and induces the signaling pathways of phytohormones, directly activating plant defenses against stress [14, 25].
As a sub-tropical fruit with significant business value in the world market, grapefruit (Citrus paradisi Macf.) is primarily consumed fresh. Carboxymethylation, a modification that improves the solubility of chitosan to produce water-soluble carboxymethyl chitosan (CMCS), is promising for its application in protecting fruit injury and damage. It can enhance resistance to postharvest diseases like blue and green mold in citrus fruits caused by fungi such as ‘P. expansum and P. digitatum’ by activating specific transcription factors (TFs), particularly from the AP2/ERF, WRKY, DREB, and MYB families activating hormonal signaling pathways can operate the ROS scavenging system. Increased expression of the genes involved in ABA metabolism boosted the activation of antioxidants and delaying senescence [10]. Additionally, the immediate post-harvest induction of CMCS altered enzymes related to the antioxidant mechanism and AsA-GSH cycle in the case of grapefruit [14, 25].
The cell wall is a strong barrier against pathogens and a key defense mechanism in post-harvest fruit. The cell wall’s polysaccharides, such as pectin, cellulose, and hemicellulose, create a network that gives mechanical strength to fruit tissue can be degraded, and loss of firmness during storage can be suppressed Using CMCS with significantly improved levels of GSH and AsA, However, CMCS served as an elicitor of postharvest enhancement of cell wall protection and antioxidant function needs more investigation and exploration [14, 25].
Carboxymethyl cellulose
Edible coatings as polysaccharides are applied to products to preserve quality by controlling physiological, biochemical, and oxidation processes, protecting agricultural items from physio-chemical and biological issues incorporated with active ingredients, flavourings, and colorings to enhance fruit’s nutritional and sensory attributes. Carboxymethyl cellulose is a high molecular weight compound made up of glucopyranosyl units. It enhances the strength and structure of edible coatings, making them more stable and durable, and widely used in the food industry. These coatings also delay the ripening process in climacteric fruits as protective barriers against gas exchange with the environment, adjusting oxygen and water transfer rates [20] [13, 26].
Aonla fruit improvements in preservation, when coated with a 1% CMC solution effectively, reduced the levels of H2O2, MDA, and superoxide anions in the treated fruits exhibited enhanced activities of SOD, APX, POD, and CAT, alongside the attributes related to the ASA-GSH cycle. Moreover, the coated fruits showed the highest amount of total phenolic and flavonoid contents indicated with increased phenylalanine ammonia-lyase (PAL), reduced polyphenol oxidase (PPO) showed anti-browning activities. Developing a CMC-based protective layer effectively delayed chlorophyll degradation by suppressing the activities of degrading enzymes named chlorophyllase-phytyl (Chl-Phy) and chlorophyll peroxidase (Chl-POD) enzymes [13, 26].
GABA (γ-aminobutyric acid)
(GABA) γ-aminobutyric acid is notable for its extensive literature connecting it to crucial jobs in plant stress physiology. GABA links carbon and nitrogen metabolic fluxes through the GABA shunt and influences the stress response pathways indirectly influencing ROS homeostasis by interacting with polyamines (PAs). Under stress conditions leading to an elevated NADH/NAD+ ratio, GABA can be catabolized to γ-hydroxybutyrate (GHB) may mitigate excessive ROS accumulation by supplying NADPH via the action of γ-hydroxybutyrate dehydrogenase (GHBDH), supporting detoxifying enzymes, including those in the ascorbate/glutathione cycle. Proline metabolism, closely linked with GABA’s, is also activated under stress and can be converts to glutamate by ProDH and P5CR, which can be converted to GABA is further supported by enzymatic and nonenzymatic reactions, emphasizing the intricate metabolic networking essential for plant stress adaptation (Table 1) [24,25,26].
The function of MIOX, a key enzyme in the myo-inositol pathway, in AsA biosynthesis remains to be determined. Various responses of PpMIOX4 transcripts to GABA treatment suggest the need for further investigation into how PpMIOX4 influences AsA biosynthesis in peach fruits [27]. GABA treatment also significantly improved the expression of all three GSH biosynthetic genes -PpGR2, PpDHAR2, PpMDHAR, PpGPX2, and PpAPX of the AsA-GSH cycle in peaches, promoting GSH accumulation. The ethylene-responsive factor (ERF) subfamily, which constitutes the most significant group within the plant AP2/ERF superfamily, has been extensively studied for its roles in conferring resistance to various abiotic stresses such as drought, salinity, and chilling [28] by controlling several metabolic pathways in Arabidopsis, tomato, and citrus. The GABA induced the expression of PpERF in fruits, suggesting a potential role of ERFs in activating AsA and GSH [29].
Hydrogen sulfide
In plant cells, hydrogen sulfide (H2S) is found in various subcellular compartments associated with enzymes involved in sulfur metabolism. The chloroplast helps in the sulfate assimilation by hosting sulfite reductase, which catalyses sulfite reduction to sulfide. Alternative pathways for sulfide generation exist in the cytosol and mitochondria. In the cytosol, H2S can be unfolded from cysteine through the action of enzymes like ‘D-cysteine desulfhydrase (DCD) and L-cysteine desulfhydrase (LCD)’, resulting in the production of ammonia and pyruvate. Meanwhile, in the mitochondria, β-cyanoalanine synthase facilitates H2S production by converting cyanide to β-cyanoalanine, utilizing cysteine. Accumulating evidence highlights H2S as a significant signaling molecule in plant-environment interactions. H2S interacts with the oxidative stress response network involving ROS at different levels, including the modulation of ROS-processing systems through changes in gene expression and protein modifications. The crosstalk between H2S and ROS also involves factors like nitric oxide, influencing crucial cellular processes. Treatment with H2S has been shown to increase the activities of several enzymes including APX, DHAR, GR, SOD, and CAT in the AsA-GSH pathway [12,27,28].
Hydrogen sulfide-applied banana fruits exhibited augmented proline (An essential osmolyte) levels and related enzyme activities [28]. Hydrogen sulfide treatment increased P5CS and OAT activities and decreased PDH a rate-limiting enzyme of proline degradation activity, leading to elevated proline levels in the fruits during chilling stress. Similarly, hydrogen sulfide fumigation led to higher endogenous GABA levels and showed increased total phenolic content, attributed to decreased activity of PPO, increased PAL activity evidenced reduced browning. Furthermore, treated banana plants demonstrated elevated activities of key antioxidant enzymes. It synergistically regulates plant energy metabolism through enzymes such as H+-ATPase, Ca2+-ATPase, and cytochrome C oxidase [30], resulting in delayed cellular energy loss and significantly improved chilling tolerance of banana fruits. The breakdown of chlorophyll involves specific chlorophyll catabolism enzymes. In contrast, hydrogen sulfide fumigation markedly inhibited these enzymes, thereby preserving the greenness of banana peels under postharvest chilling stress [31]. Phenolic compounds are known for their ROS scavenging potential, reducing oxidative stress and attenuating membrane lipid peroxidation, thus enhancing Chilling Injury (CI) tolerance. Hydrogen sulfidic fumigation of banana fruits substantially reduced POD and PPO activities with increased PAL activity, leading to higher phenolic content, suggesting effectively mitigates CI in bananas stored under cold conditions (Figure 1 and Table 1) [12].
Ozone
Ozone (O3), a triatomic form of oxygen, forms when molecular oxygen (O2) reacts with an oxygen-free radical. It has a characteristic pungent smell and appears as a blue gas when generated from dry air, although it is colorless when derived from high-purity oxygen. In typical concentrations used for food processing, its color is not detectable. Ozone has a relatively high oxidation-reduction potential and is nearly 13 times more soluble in water than oxygen, making it a potent oxidizing agent that rapidly decomposes back to atmospheric oxygen, leaving no residues. Its antimicrobial activity results from direct interactions (ozone molecules) and indirect effects (free radicals). In addition to its antimicrobial properties, ozone can enhance plant stress tolerance by stimulating cellular ROS scavenging systems, thus boosting the synthesis of antioxidant enzymes. Ozone treatment influences different reactions by enhancing antioxidant enzyme activities, including POD, superoxide dismutase (SOD), and PAL while inhibiting PPO activity. Furthermore, the efficacy of O3 in maintaining the quality of minimally processed products is evident through its influence on total soluble solid (TSS) content and titratable acidity (TA). Overall, Ozone acts as a signal to induce rapid defensive responses in plants, activating multiple pathways that enhance their capability to reduce free radicals, thereby prolonging the freshness of postharvest vegetables [32-34].
Ozone treatment improves postharvest storage of cantaloupe by enhancing antioxidant capacity, particularly in the ASA-GSH cycle by reducing H2O2 and MDA accumulation. Additionally, mechanical processing can accelerate senescence and decay in red pitaya. Overall, low-concentration, long-term ozone treatment can delay aging and preserve the quality of fruits [35].
Methyl jasmonate
Jasmonates (JAs) are cyclopentanone components or plant hormones derived from α-linolenic acid. JA, including its derivatives like methyl jasmonate (MJ), forms a set of oxygenated fatty acids and is a critical stimulator in their biosynthesis. JA is synthesized from fatty acids in three steps, starting in the chloroplast membrane. Key enzymes related in this pathway include lipoxygenase (LOX), allene oxide synthase (AOS), allene oxide cyclase (AOC), hydroperoxide lyase (HPL), alcohol acyltransferase (AAT), and alcohol dehydrogenase (ADH), which together facilitate the synthesis of jasmonic acid and its volatile organic compounds (VOCs) in plant cells [33]. Jasmonates are essential for mediating plant's biotic and abiotic stresses. This interaction triggers a series of defense responses, including the production of ROS and reactive nitrogen species (RNS) and the induction of enzymes that protect against oxidative stress also triggers the synthesis and build-up of signaling molecules like JA, SA, NO, and ET within the cell, thereby influencing the expression of genes related to secondary metabolite production. Nevertheless, MeJA is the most widely used elicitor perceived by specific receptors involved in signal transduction [33,34]. Variations in the expression of genes encoding key enzymes directly influence the accumulation of corresponding secondary metabolites, with PAL regulation, MEP (methylerythritol phosphate), and MVA (mevalonate) pathways seeing increased expression with MeJA treatment [36, 37].
GC-MS analysis indicated that fruits at the complete red stage contained more types and higher concentrations of VOCs compared to those at the early ripening stage. Notably, many VOCs are derived from the LOX pathway. Moreover, the content of VOCs increased with the rise in ethylene production. Correlation analysis showed a strong positive relationship between VOC content and ethylene production, suggesting that ethylene is a significant mediator in VOC biosynthesis. The application of 1-methylcyclopropene (1-MCP) appeared to decrease the accumulation of VOCs by inhibiting ethylene production, whereas MeJA treatment enhanced VOC accumulation by stimulating ethylene production. Additionally, it was found that MeJA treatment promoted VOC accumulation and regulated the antioxidant system, including the ascorbate-glutathione (AsA-GSH) cycle [1].
Researchers are increasingly focusing on the ICE-CBF-COR signaling pathway, recognized as a critical component of the cold acclimation process. Inducer of CBF Expression (ICE), a MYC-type basic helix-loop-helix (bHLH) family transcription factor, plays a central role in the induction of C-repeat binding factors (CBFs) during cold exposure. This pathway is essential for activating the expression of downstream genes encoding osmoregulatory substances. Although the ICE-CBF-COR pathway is pivotal in plant freezing tolerance, current research also examines the influence of hormones on cold stress responses. Essential hormones such as ABA, GA, BRs, JA, auxin, CK, melatonin, and polyamines impact CBF expression [38]. MeJA-treated fruits exhibited significantly increased expression levels of the C-repeat-binding factor 1 (SlCBF1), a cold-regulated gene (SlCOR413), and inducers of SlCBF expression (SlICE1 and SlICEa), all components of the SlICE-SlCBF-SlCOR (ICC) signaling pathway.
Additionally, correlation analysis suggested that genes involved in the AsA-GSH cycle and the ICC signaling pathway were closely associated with the transcription of SlMYC2, a jasmonic acid signaling transcription factor. These findings underscore the role of MeJA-mediated regulation of the AsA-GSH cycle and the ICC signaling pathway during cold tolerance in tomato fruits (Figure 1) (Table 1) [10, 11].
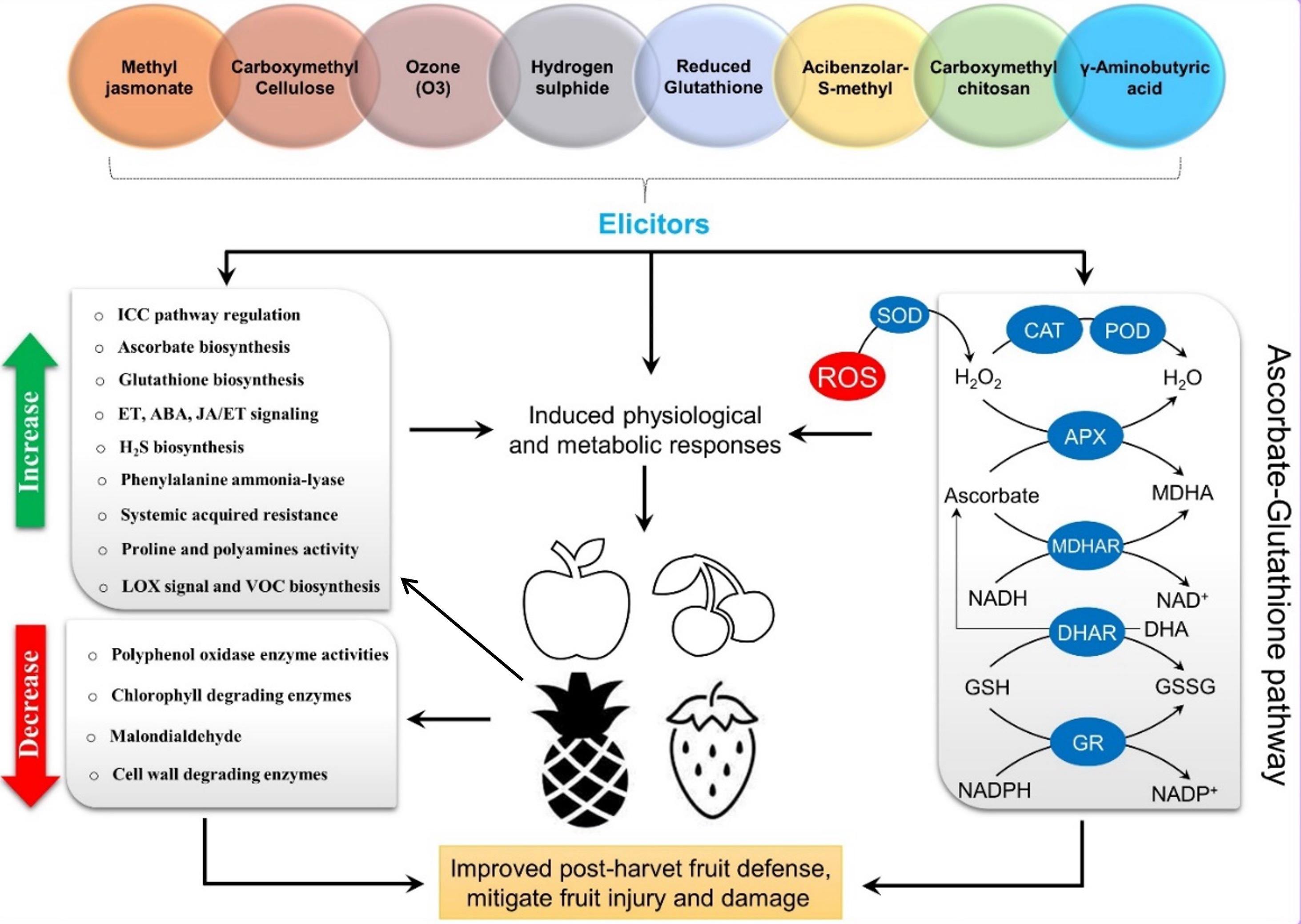
Table 1. Role of elicited ASA-glutathione pathway in postharvest stress mitigation in fruit.
CONCLUSION AND FUTURE PROSPECTS
Systemic acquired resistance (SAR) is a key trait for protecting plants or fruits from pathogen attacks. Several secondary metabolites and amino acids including carotenoid biosynthesis, accumulation of flavonoids, carotenoids, and ascorbic acid in response to elicitors are crucial. Accumulation of these metabolites along with boost-up antioxidant activity through the AsA –GSH cycle in harvested fruit, scavenges ROS maintains cellular integrity, and declines lipid peroxidation. Furthermore, the alteration of a series of metabolic changes stabilizes membrane phospholipid metabolism and maintains cell membrane potential and fluidity, which helps to preserve postharvest fruit quality. Importantly, the elicitor responses delay fruit ripening by suppressing ethylene production and respiration rates that lead to maintaining sugar levels, organic acids, and firmness during storage. Postharvest elicitor responsiveness provides a simple and cost-effective strategy with plant defense for managing harvested fruit's abiotic and biotic stresses. Additionally, the efficacy of elicitors may vary based on fruit species or cultivars, elicitor type, concentration, and timing. Agricultural practices such as fertilization, irrigation, and pruning also influence the effectiveness of elicitors. Furthermore, the application technique—including spray density and intensity—and the equipment used are critical factors. Postharvest ecological conditions, including storage temperature, relative humidity, and gas composition, also affect elicitor performance, and optimal combinations of these factors must be determined. Despite extensive research into the effects of postharvest elicitor spray on fruit properties and a preliminary understanding of the physiological and cytological mechanisms, deeper mechanisms remain elusive. Advanced multi-omic approaches and gene-editing approaches are necessary for improving fruit shelf-life, and storage duration and mitigating stress-induced damages. Moreover, elicitors-induced accumulation of various natural plant metabolites and amino acids offer safer alternatives to using chemical fungicides and lower risk of resistance development. Future research must disclose the strengthening of the linkage between pre- and postharvest periods and broaden the scope of postharvest studies by application of different combined elicitors to gain rapid effects like synthetic compounds. Fruits and other edible organs provide numerous fruitful nutrients that enrich our lives and society. Unfortunately, around 33% of harvested fruits and other edible products are never consumed due to short-shelf-life, and not well-equipped storage system that causes post-harvest waste and economic loss. However, several breeding strategies can retain desirable features by declining post-harvest damages and increasing plant Defense and fruit shelf-life. Considering the demand for valuable fruits, vegetables and other edible crops new gene editing tool would be promising and effective for fruits and other edible parts improvement by improving desirable traits. This editing approach may improve traits related to fruit quality, shelf-life, yield, and post-harvest losses of fruits. Furthermore, CRISPR–Cas9 method would be a promising tool for alternative solutions of improving traits by reducing post-harvest waste and economic loss of fruits.
ACKNOWLEDGEMENTS
None.
AUTHOR CONTRIBUTIONS
MI and SMA: research concept initiation and first draft writing. MEJ: data collection and curation. AKD: figure drawing. SS, MMR, and IHS: writing, review, and editing. SMA: coordinated the whole work.
CONFLICTS OF INTEREST
There is no conflict of interest among the authors related to this work.
References
- [1]Min D, Li Z, et al. The co-regulation of ethylene biosynthesis and ascorbate–glutathione cycle by methy jasmonate contributes to aroma formation of tomato fruit during postharvest ripening. Journal of Agricultural and Food Chemistry. 2020;68:10822-32.
- [2]Huang R, Cheng Y, et al. Postharvest application of acibenzolar- s-methyl delays the senescence of pears by mediating the ascorbate-glutathione cycle. Scientia Horticulturae. 2022;293:110741.
- [3]Iñiguez-Moreno M, González-González RB, et al. Nano and technological frontiers as a sustainable platform for postharvest preservation of berry fruits. Foods. 2023;12:3159.
- [4]Palumbo M, Attolico G, et al. Emerging postharvest technologies to enhance the shelf-life of fruit and vegetables: An overview. Foods. 2022;11:3925.
- [5]Rodrigues M, Ordoñez-Trejo EJ, et al. Dissecting postharvest chilling injuries in pome and stone fruit through integrated omics. Frontiers in Plant Science. 2024;14.
- [6]Zhang W, Jiang Y, et al. The role of different natural organic acids in postharvest fruit quality management and its mechanism. Food Frontiers. 2023;4:1127-43.
- [7]Cuéllar-Torres EA, Aguilera-Aguirre S, et al. Molecular aspects revealed by omics technologies related to the defense system activation in fruits in response to elicitors: A review. Horticulturae. 2023;9:558.
- [8]Gong D, Bi Y, et al. Preharvest elicitors spray improves antioxidant activity, alleviates chilling injury, and maintains quality in harvested fruit. Horticulturae. 2022;8:1208.
- [9]Zhou Y, Liu J, et al. Exogenous glutathione maintains the postharvest quality of mango fruit by modulating the ascorbate-glutathione cycle. PeerJ. 2023;11:e15902.
- [10]Li Z, Min D, et al. The roles of slmyc2 in regulating ascorbate-glutathione cycle mediated by methyl jasmonate in postharvest tomato fruits under cold stress. Scientia Horticulturae. 2021;288:110406.
- [11]Zhu L, Yu H, et al. Effect of methyl jasmonate on the quality and antioxidant capacity by modulating ascorbate-glutathione cycle in peach fruit. Scientia Horticulturae. 2022;303:111216.
- [12]Ali S, Nawaz A, et al. Hydrogen sulfide mitigates chilling injury of postharvest banana fruits by regulating γ-aminobutyric acid shunt pathway and ascorbate–glutathione cycle. Frontiers in Plant Science. 2022;13.
- [13]Ali S, Nawaz A, et al. Carboxymethyl cellulose coating maintains quality of harvested aonla fruit by regulating oxidative stress and ascorbate-glutathione cycle. Postharvest Biology and Technology. 2024;207:112621.
- [14]Wu H, Wang F, et al. Carboxymethyl chitosan different durations induces disease resistance of grapefruit by modulating ascorbate-glutathione cycle and cell wall metabolism. Postharvest Biology and Technology. 2024;211:112845.
- [15]Lu X, Zhang H, et al. Effects of ozone treatment on gene profiling involved in asa-gsh cycle in postharvest cantaloupe. Scientia Horticulturae. 2023;312:111843.
- [16]Yao M, Ge W, et al. Exogenous glutathione alleviates chilling injury in postharvest bell pepper by modulating the ascorbate-glutathione (asa-gsh) cycle. Food Chemistry. 2021;352:129458.
- [17]Imahori Y, Bai J. Postharvest management of fruits and vegetables—series ii. Foods. 2024;13:1049.
- [18]Meitha K, Pramesti Y, et al. Reactive oxygen species and antioxidants in postharvest vegetables and fruits. International Journal of Food Science. 2020;2020:8817778.
- [19]Rajput VD, Harish, et al. Recent developments in enzymatic antioxidant defence mechanism in plants with special reference to abiotic stress. Biology. 2021;10:267.
- [20]Hasanuzzaman M, Bhuyan MHMB, et al. Regulation of ascorbate-glutathione pathway in mitigating oxidative damage in plants under abiotic stress. Antioxidants. 2019;8:384.
- [21]Zheng X, Gong M, et al. Metabolism and regulation of ascorbic acid in fruits. Plants. 2022;11:1602.
- [22]Hasanuzzaman M, Nahar K, et al. Glutathione in plants: Biosynthesis and physiological role in environmental stress tolerance. Physiology and Molecular Biology of Plants. 2017;23:249-68.
- [23]Humbal A, Pathak B. Influence of exogenous elicitors on the production of secondary metabolite in plants: A review (“vsi: Secondary metabolites”). Plant Stress. 2023;8:100166.
- [24]Wang B, Bi Y. The role of signal production and transduction in induced resistance of harvested fruits and vegetables. Food Quality and Safety. 2021;5.
- [25]Ji H, Wang J, et al. Meta-analysis of chitosan-mediated effects on plant defense against oxidative stress. Science of The Total Environment. 2022;851:158212.
- [26]Panahirad S, Dadpour M, et al. Applications of carboxymethyl cellulose- and pectin-based active edible coatings in preservation of fruits and vegetables: A review. Trends in Food Science & Technology. 2021;110:663-73.
- [27]Celi GEA, Gratão PL, et al. Physiological and biochemical roles of ascorbic acid on mitigation of abiotic stresses in plants. Plant Physiology and Biochemistry. 2023;202:107970.
- [28]Wu Y, Li X, et al. Erf subfamily transcription factors and their function in plant responses to abiotic stresses. Frontiers in Plant Science. 2022;13.
- [29]Zhou C, Dong W, et al. Γ-aminobutyric acid treatment induced chilling tolerance in postharvest peach fruit by upregulating ascorbic acid and glutathione contents at the molecular level. Frontiers in Plant Science. 2022;13.
- [30]Yang Z, Zhu S, et al. Nitric oxide modulates folate-mediated one-carbon metabolism and mitochondrial energy levels of peaches during cold storage. Frontiers in Nutrition. 2023;10.
- [31]Anwar A, She M, et al. Biological roles of ornithine aminotransferase (oat) in plant stress tolerance: Present progress and future perspectives. International Journal of Molecular Sciences. 2018;19:3681.
- [32]Botondi R, Barone M, et al. A review into the effectiveness of ozone technology for improving the safety and preserving the quality of fresh-cut fruits and vegetables. Foods. 2021;10:748.
- [33]Aslam R, Alam MS, et al. Sanitization potential of ozone and its role in postharvest quality management of fruits and vegetables. Food Engineering Reviews. 2020;12:48-67.
- [34]Vijay Rakesh Reddy S, Sudhakar Rao DV, et al. Role of ozone in post-harvest disinfection and processing of horticultural crops: A review. Ozone: Science & Engineering. 2022;44:127-46.
- [35]Li C, Tao J, et al. Gaseous ozone regulates reactive oxygen species metabolism and ascorbate–glutathione cycle to delay the senescence of fresh-cut red pitaya (selenicereus undatus) fruit. Scientia Horticulturae. 2023;312:111839.
- [36]Cook R, Lupette J, et al. The role of chloroplast membrane lipid metabolism in plant environmental responses. Cells. 2021;10:706.
- [37]Ho T-T, Murthy HN, et al. Methyl jasmonate induced oxidative stress and accumulation of secondary metabolites in plant cell and organ cultures. International Journal of Molecular Sciences. 2020;21:716.
- [38]Ritonga FN, Chen S. Physiological and molecular mechanism involved in cold stress tolerance in plants. Plants. 2020;9:560.