Nitric oxide facilitates the activation of iron acquisition genes in soybean (Glycine max L.) exposed to iron deficiency
Abstract
Glycine max L.). Fe-deficiency causes chlorosis, growth retardation, low photosynthetic performance, and productivity. Nitric oxide (NO) is known to serve as a signalling molecule in plants, but its role in mitigating Fe-deficiency in soybeans is still vague. Therefore, the study aims to explore physiological and molecular mechanisms associated with Fe-acquisition and homeostasis is highly demandable. In this study, we found Fe-deficiency occurred leaf chlorosis, inhibiting photosynthetic performance and biomass yield in soybean. However, the exogenous supplementation of sodium nitroprusside, a donor of nitric oxide (NO) significantly restored these physiological attributes. The fluorescence intensity of NO indicates that NO-singling induced in response to Fe-deficiency, along with NO induces conversion of Fe3+ to Fe2+, which leads to Fe acquisition and homeostasis mechanism in Strategy I soybean plants. This study further suggests that NO up-regulates the expression candidate genes ZmIRT1, ZmFRO2, and ZmSultr1;3 related to Fe-acquisition and homeostasis in soybean plants. These findings might be useful to soybean breeders and farmers for coping with Fe-deficiency in Strategy I soybean and other grains crops.
INTRODUCTION
Iron (Fe) is a microelement crucial for many physiological processes such as photosynthesis, respiration, DNA synthesis, and nitrogen fixation in plants [1]. Fe-limitation causes leaf chlorosis, plant growth inhibition, mineral shortage, and plant productivity loss in plants including legumes [2]. Fe-deficiency limitation is very common in alkaline soils as well as soils possessing high cation exchange capacity [3]. In high pH soils, the deposition of CaCO₃ forms bicarbonate (HCO⁻₃) suffers system due to inhibition and/or poor aeration that may lead to Fe-shortage in plants [4]. In addition, calcareous soil combined with overwatering initiates Fe deficiency in plants [2]. Fe is essential for successful legume-rhizobium symbiosis. Fe is required for leghemoglobin protein to carry oxygen in cells, for nitrogenase enzyme, and for cytochromes-involving electron transport system (ETS) in bacteroids [5].
Plants acquire Fe from soils through Strategy-I(dicot and non‐graminaceous species) and strategy-II mechanisms (graminaceous species) [6]. These complex processes allow plants for Fe acquisition and homeostasis through a series of physiological and molecular alterations. In the Fe acquisition process, ferric (Fe3+) converts into ferrous (Fe2+) presence of ferric chelate reductase (FCR) enzyme that is one of the key mechanisms of Strategy-I plants [7]. Another way is to solubilization of Fe in soils by secreting H+ into the rhizosphere [2]. In addition, Fe transporters play a vital role at transcriptional and posttranscriptional levels for uptalking Fe from soils [8]. Iron-regulated transporter1 (IRT1) is a key transporter gene that uptake Fe2+ in Strategy-I plants [4]. The ferric reduction oxidase 2 (FRO2) coordinates IRT1 responsible for high-affinity Fe uptake from soils [8].
Signaling molecules are involved in regulating the adaptive response of Fe in plants. Nitric oxide (NO) is a gracious molecule that regulates physiological processes and stress tolerance in plants [9]. NO signaling is involved in Fe-deficiency response and homeostasis in plants [10]. Numerous studies have shown that NO is the key marker of Fe-deficiency response that coordinates the expression of genes and transcription factors related to Fe-regulation in several plant species [4, 10, 11]. In this scenario, the role of NO in recovering iron deficiency and the response of Fe-responsive candidate genes in legume crops could play a significant role in nutritional security.
Soybean (Glycine max L.) is an important legume and an excellent source of food, feed, and promising biofuel. Fe deficiency is a major limitation in high pH calcareous soils, The Fe-shortage problem leads to a decline in soybean yield equivalent economic loss of $820 million per year [12]. Therefore, eco-friendly, and cost-effective strategies and the discovery of molecular alteration associated with Fe acquisition and homeostasis of grain crops are highly demandable. In this context, we have explored mechanistic insights into NO-mediated regulation of key genes associated with Fe-acquisition and homeostasis in soybean plants.
MATERIALS AND METHODS
Plant cultivation with Fe-deficiency and NO treatment
Disease-free-viable soybean (Glycine max L.) seeds were surface sterilized with 70% ethanol for 1 min, seeds were washed properly using double distilled water, and then seeds were transferred to a germination tray for three days. Subsequently, 3 days later the soybean’s small seedlings were transferred to micro-macro nutrient-containing traditional Hoagland solution [13]. The soybean seedlings were grown with the following combinations: 25 µM FeNaEDTA with all macro-micro element containing media (control); only 0.1 µM FeNaEDTA (−Fe); 0.1 µM FeNaEDTA +sodium nitroprusside (100 µM) as nitric oxide (NO) donor (-Fe+NO); and 100 µM SNP treatment (NO). All plants were maintained in a controlled environment with 60–65% relative humidity (RH), 200 μmolm−2 s−1 light intensity, temperature 25 °C, and 14 h light and 10 h dark combination. The treatments were terminated after two weeks. Samples were collected with liquid N2, and then frozen samples were stored at -80°C until further analyses. At least three biological replications were considered for each treatment.
Determination of photosynthetic parameters and plant biomass
The treated plants were dark adapted for 1 h prior to measuring Fv/Fm and SPAD value (leaf greenness). The maximum yield of photosystem II (Fv/Fm) was measured using a portable fluorometer system (PAM 200, Effeltrich, Germany), while SPAD score was recorded using a SPAD meter (Minolta, Japan). Soybean seedlings were dried at 70°C for 72 h and then weighted using an electrical balance (Mettler PM 200, Switzerland). The mean value of three independent seedlings was graphically presented for each treatment.
Measurement of Fe and S in plants
Soybean leaf samples were dried at 70°C for 72 h and then digested using an acid solution (HClO4/HNO3; 1:3 v/v), the Fe and S concentrations were determined in plant sample using ICP-MS system (Agilent 7700, Santa Clara, CA, USA). A standard curve was fit using a multi-element ICP-standard-solution (ROTI®STAR, Roth, Germany).
Fluorescent histochemical staining of NO in soybean root cells
The nitric oxide (NO) signal was measured using a Fluorescence system (CLS-01-00076, Logos Biosystem, South Korea). NO-specific fluorescent probe 4,5-diaminofluorescein diacetate (DAF-2DA) (Sigma-Aldrich, Burlington, MA, USA) was used for fluorescent intensity. In brief, the control and treated soybean root tips were washed with deionized water properly to remove excess nutrients from the root tip surface, excised them abound 2 mm in size, the excised root tips were incubated 10 mM Tris−HCl buffer (pH 6.5) containing 10 μM DAF-2DA at dark condition for 30 min. Subsequently, the root tips were washed trice with diethylpyrocarbonate (DEPC) treated water. The reaction of the NO signal with DAF-2DA was observed with 495 nm excitation and 515 nm emissions.
Expression analysis of key genes related to Fe-acquisition and homeostasis
Total RNA was isolated from soybean samples using an RNA extraction kit (QIAGEN, Hilden, Germany). Briefly, 0.1 g of plant tissue was homogenized with extraction buffer containing 2M DDT and 1% (v/v) β-ME. The whole procedure associated with RNA extraction, cDNA synthesis, and quantitative real-time polymerase chain reaction (qRT-PCR) was performed according to the method used previously [14]. The ZmActin was used as internal control, and the expression levels of key genes were calculated using the 2−ΔΔCt method [15].
RESULTS
Changes in plant morphology, photosynthesis, and plant biomass
Soybean plants showed significant chlorosis in response to Fe-deficiency (Figure 1). As a consequence, nutrient shortage, photosynthesis efficiency, pigment content, and biomass yield significantly decreased in response to Fe-deficiency (Figure 2A-C). Furthermore, photosynthetic parameters including Fv/Fm and SPAD score were significantly lower under Fe-deficiency compared to control plants (Figure 2 A, B). The plant biomass significantly declined under Fe-deficiency (Figure 2C). Interestingly, the supplementation of NO significantly restored these limitations after adding to the Fe-deficient condition, suggesting NO is a potent regulator that is involved in recovering Fe-deficiency in soybean plants.
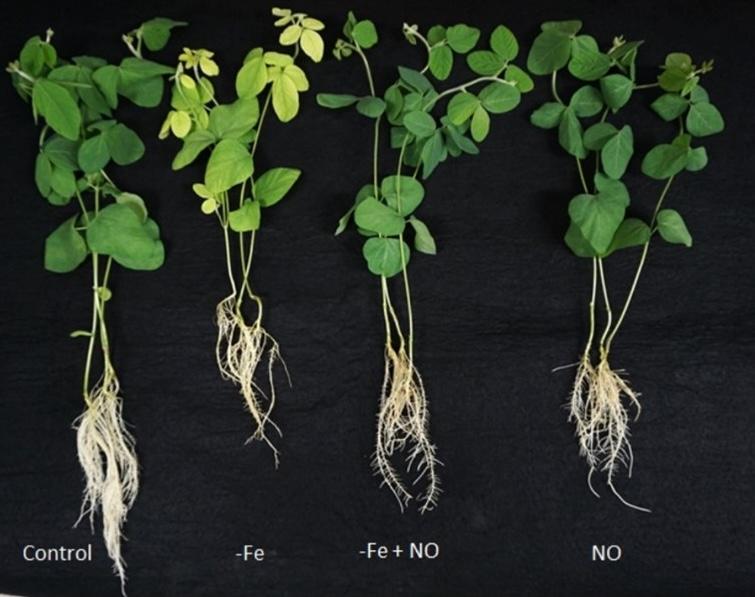
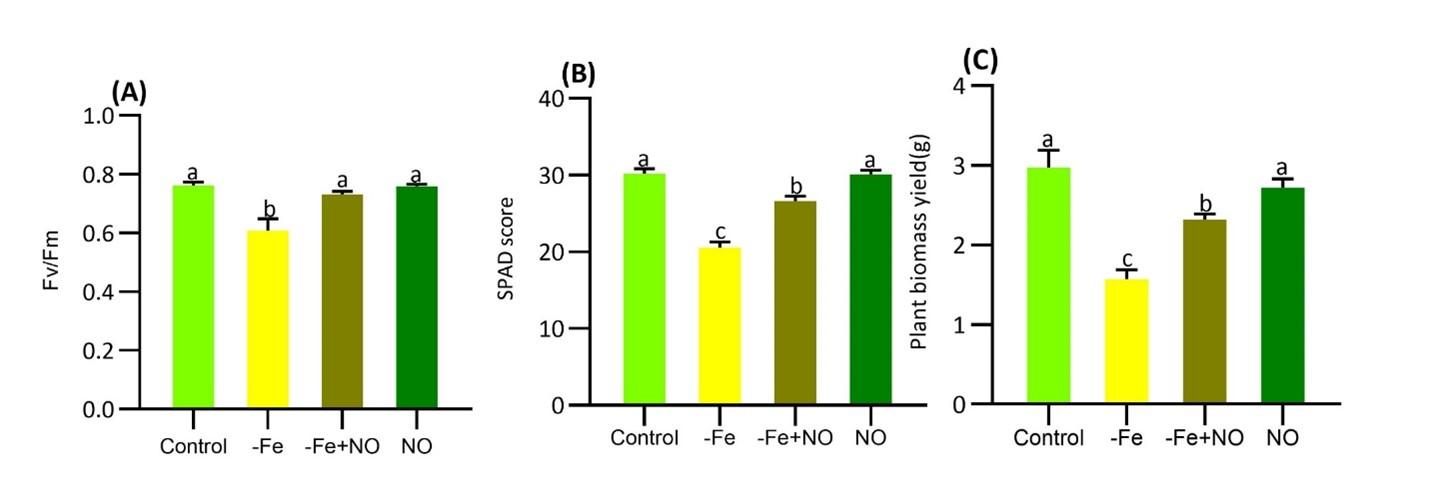
Regulation of Fe and S concentration
Fe-deficiency drastically reduced Fe and S concentrations in soybean leaves compared to the control (Figure 3). However, NO supplementation significantly recovered the acquisitions of both elements in soybeans (Figures 3A, B). NO application to the control plants slightly enhanced Fe and S levels, but the increases were not statistically significant compared to the control. The recovery of Fe and S concentrations in response to NO indicates that NO actively enhanced the levels of these elements, which had declined under Fe deficiency.
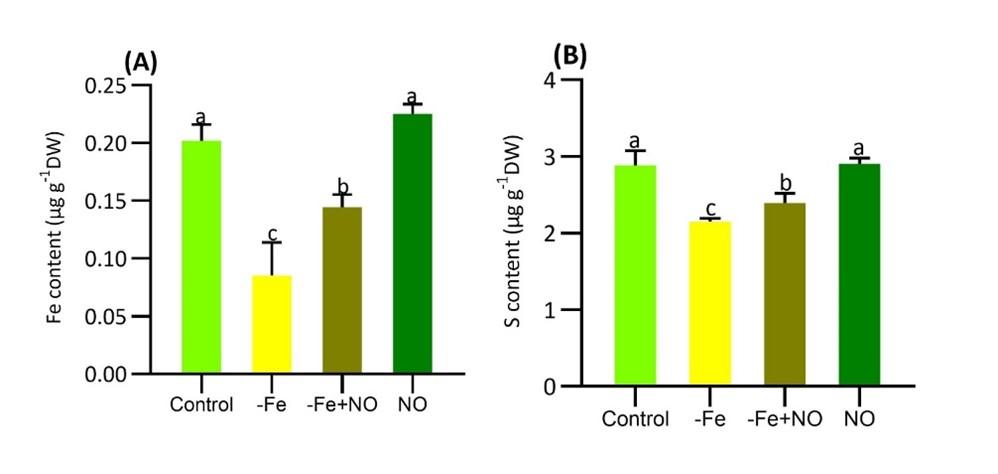
Detection of NO-signal under Fe-deficiency
The root tip of the Fe-deficient plant induced NO-signal, while a NO-responsive signal was not observed in control plants (Figure 4). The fluorescence intensity of NO was higher after exogenously supplemented NO in Fe-deficient plants which was significantly higher compared to Fe-deficient and control plants (Figure 4A, B). The fluorescence intensity was found to be induced in NO-treated positive control plants. These results indicate that NO signal is induced in response to exogenous endogenous NO in root tips cells, which indicates NO-involving Fe-adaptive mechanisms in soybean plants.
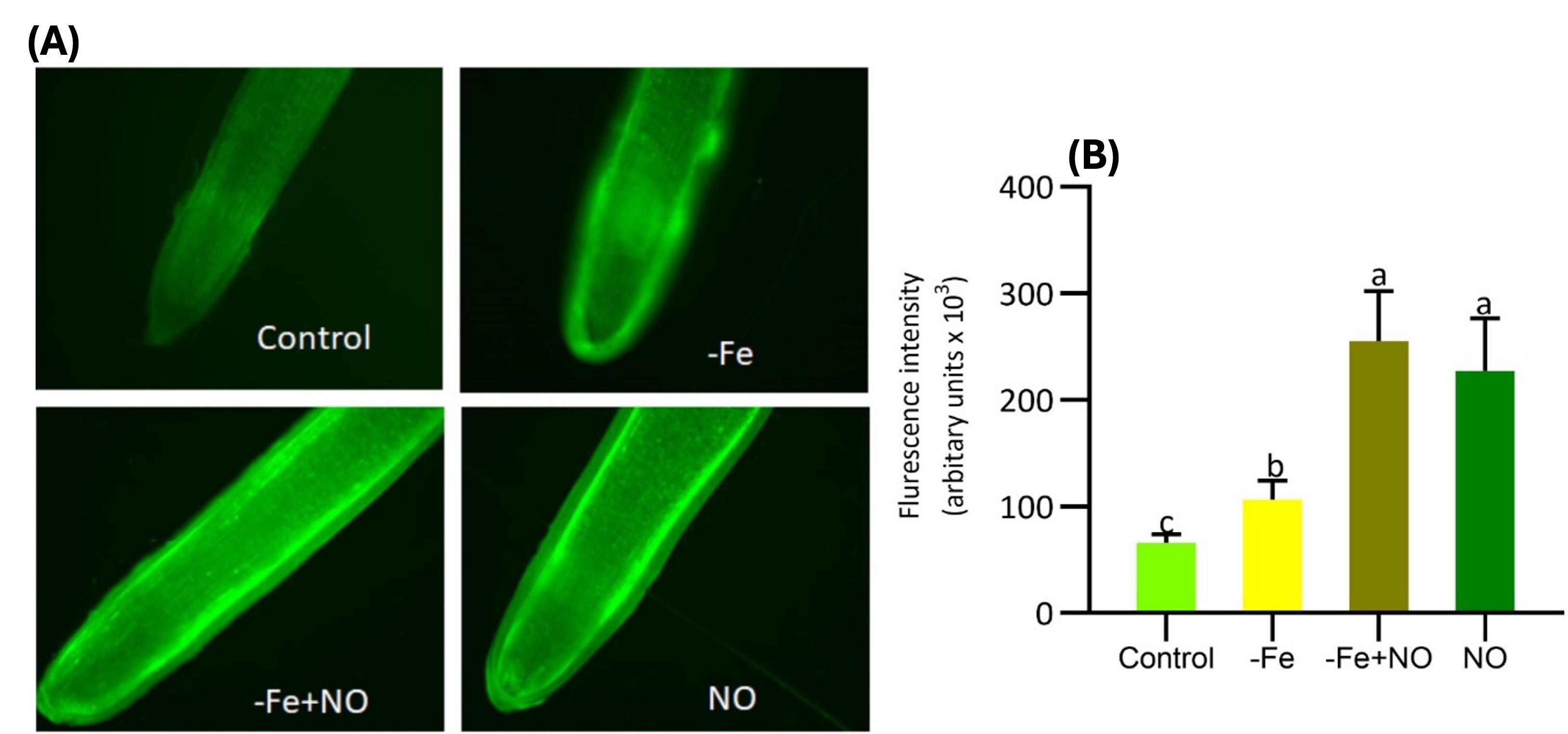
Upregulation of GmIRT1, GmFRO2 and GmSultr1;3
Several key genes related to Fe regulation and homeostasis were induced in response to Fe-deficiency (Figure 5).The expression of GmIRT1 was significantly induced by Fe-deficiency that was, even more, higher after exogenous supplementation of NO in plants (Figure 5A). Expression of low-Fe-inducible ferric chelate reductase gene GmFRO2 drastically upregulated by Fe-deficiency, indicating Fe-deficiency is significantly reduced at root cells (Figure 5B). In addition, a significant up-regulation pattern was observed for sulfur transporter gene GmSultr1;3 compared to control plants (Figure 5B). Hence, the expression of this gene was substantially expressed in response to NO-supplementation in soybean plants (Figure 5C).
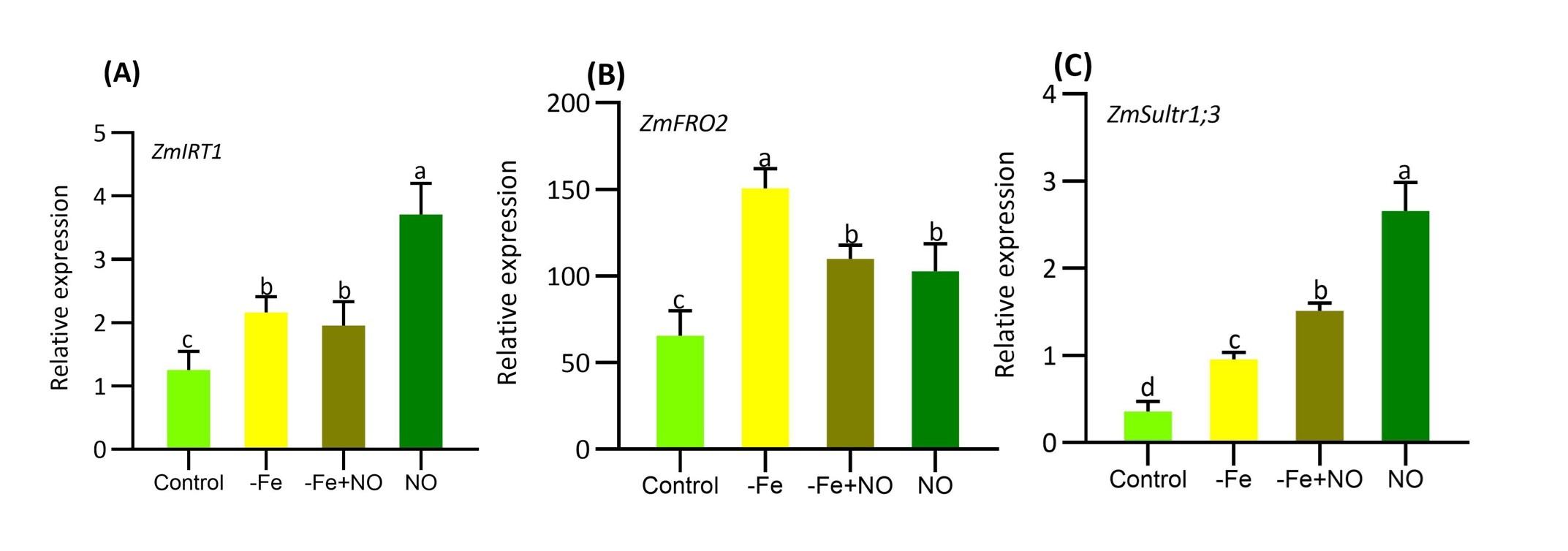
DISCUSSION
Fe-deficiency-induced leaf chlorosis is obvious in dicot plants that cause growth inhibition, reduction of Fe concentration, and plant productivity [2]. In this study, we have explored mechanistic insights of NO-mediated restoration of these attributes in soybean plants. In the following sections, we discussed the role of NO in regulating physiological and molecular mechanisms, upregulation of key genes related to Fe-acquisition and homeostasis in soybean with other Strategy I plants, and their interpretations.
Restoration of Fe, photosynthetic performance, and plant productivity in soybeans is a promising sign for Strategy I grain crops. NO plays a significant role in reactivating the process related to Fe-acquisition, enhancement of chlorophyll content, and regulation of key genes involved in Fe-regulation in plants [10]. Fe chlorosis and Fe resupply significantly regulate leaf xylem architecture, water relations, gas exchange, and stomatal performance of field-grown peach, which alters its original form and performance of photosystem II (PS-II) and influences the Fe-dependent chlorophyll biosynthesis process [16]. Another study also documented that Fe-deficiency-induced chlorotic leaf negatively regulated PS-II in plants [17]. As a consequence of these events, energy production, and biomass yield are hampered in plants. In this NO-treated soybean, the PS-II (Fv/Fm) rate significantly increased which suggests NO can overcome PS-II damage along with leaf greenness. In addition, NO signal found Fe-deficient roots, but root architecture remains unchanged and/or little improved in NO-treated plants, indicating Fe-deficiency not significantly damaged the root cells compared to the leaf in Strategy I soybean. Our findings suggest that NO can enhance Fe-acquisition and homeostasis processes in soybeans, and NO improves PS-II performance allowing them to translocate sufficient Fe content in the PS-II reaction centre in soybean plants.
Plants have evolved an adaptive response to Fe-deficiency, but this response depends on Strategy I and II mechanisms and plant species. NO restored Fe concentration in the Strategy I alfalfa legume [11]. Moreover, S directly interacts with Fe, and the capacity to uptake Fe is highly dependable on S availability in monocot and dicot plant species [18]. In our current study, the fashion of accumulation patterns of Fe and S are similar suggesting a correlation and common mechanism in Strategy I soybean plants. Hence, this accumulation was enhanced after supplementation of NO in Fe-deficient plants. The overall findings imply that NO supplementation induces Fe and S availability in soybeans and helps to restore the Fe-deficiency problem in soybeans.
The FRO2 gene encodes low-iron-inducible ferric chelate reductase enzyme responsible for the reduction of Fe status in plants, and FRO2 coordinates IRT1 that helps to Fe-uptake in Arabidopsis [8]. In this NO-treated soybean, the expression ZmFRO2 was highly expressed following the imposition of Fe-starvation plants, while ZmIRT1 and ZmSultr1;3 were highly induced in response to exogenous supplementation of NO supply. As a result, we noticed that NO may promote the conversion of Fe3+ to Fe2+, which leads to Fe acquisition and homeostasis procession in Strategy I soybean plants. This finding is supported by the observation of the Strategy-I plant where NO played as an inducer of Strategy-I responses to increase Fe availability and mobilization in Fe-starved broccoli [4]. Therefore, the above findings imply that NO-triggers the expression of key genes related to Fe acquisition and homeostasis in soybean plants.
CONCLUSION
This study implies the mechanistic basis of NO-mediated protection of Fe-deficiency in Strategy soybean plants (Figure 6). Fe-shortage induced chlorosis, declined chlorophyll content, photosynthetic performance, and plant biomass yield in soybean. However, the exogenous supplementation of NO significantly restored these physiological attributes in soybeans. This study further suggests that NO played a potential inducer of key genes (ZmIRT1, ZmFRO2, and ZmSultr1;3) related to Fe-acquisition and homeostasis in Strategy I soybean plants. Additionally, NO helps to induce the conversion of Fe3+ to Fe2+, which leads to Fe acquisition and homeostasis mechanism in Strategy I soybean plants (Figure 6). These findings encourage us to improve Strategy I soybean and other grains crops with Fe-sufficiency through breeding programs.
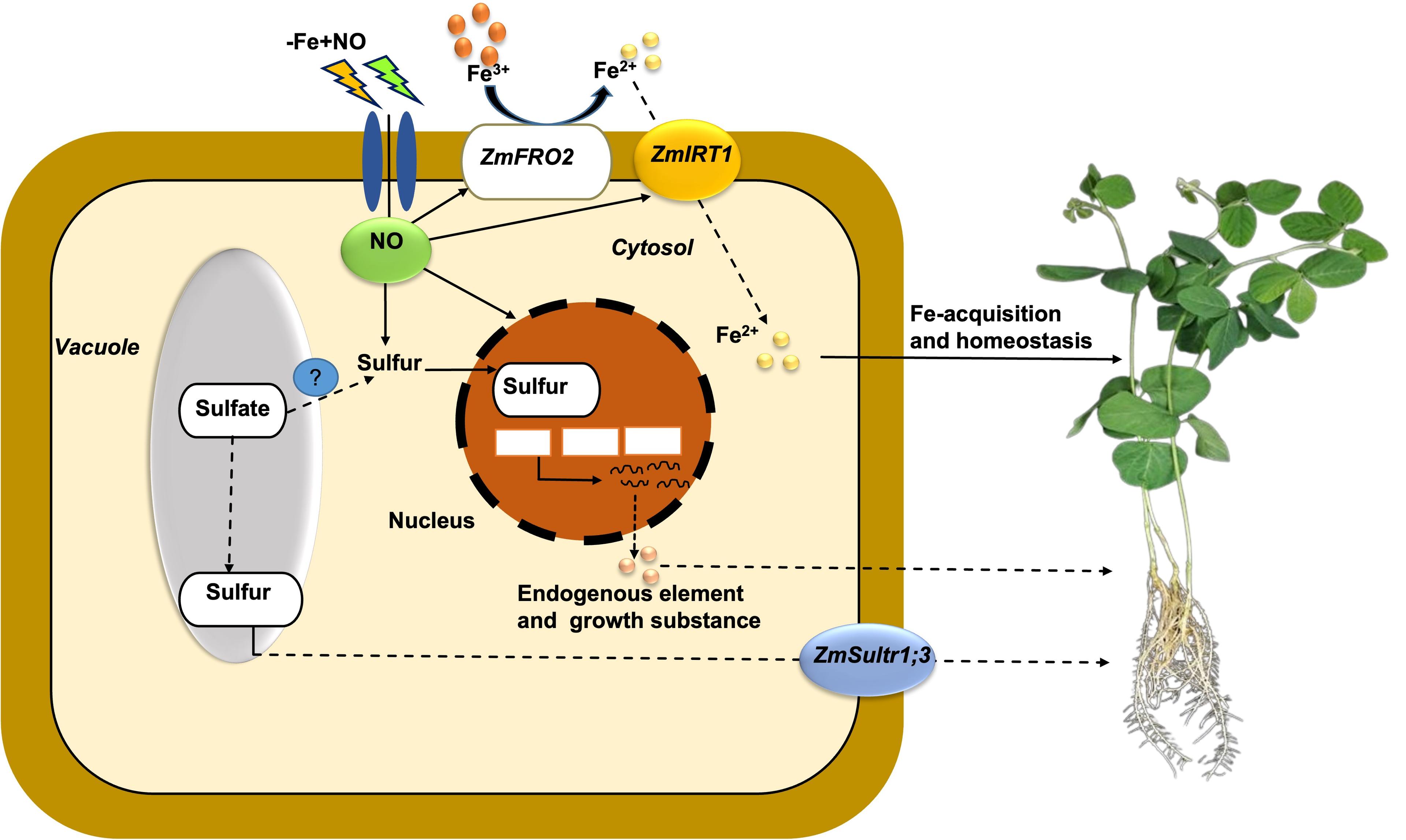
ACKNOWLEDGEMENT
We thank the Grassland and Forage Division, National Institute of Animal Science, Rural Development, Administration, Cheonan 31000, Republic of Korea for technical support.
AUTHOR CONTRIBUTIONS
RB performed plant cultivation, processed samples, and measured phenotypic data. SFD collaborated with the expression analysis of genes. SAS and MNA performed physiological observations of plants. MAR performed ICP-MS and microscopic analysis. AHK initiated the research idea and coordinated the whole work.
CONFLICTS OF INTEREST
There is no conflict of interest among the authors.
References
- [1]He X-L, Zhang W-Q, et al. Hydrogen sulfide and nitric oxide regulate the adaptation to iron deficiency by affecting Fe homeostasis and thiol redox modification in Glycine max seedlings. Plant Physiol Biochem. 2023;194:1-14.
- [2]Kabir AH, Rahman MA, et al. Mechanistic assessment of tolerance to iron deficiency mediated by Trichoderma harzianum in soybean roots. J Appli Microbiol. 2022;133:2760-78.
- [3]Rahman MA, Bagchi R, et al. Physiological and molecular characterization of strategy-I responses and expression of Fe-transporters in Fe-deficient soybean. S Afr J Bot. 2022;147:942-50.
- [4]Kabir AH, Ela EJ, et al. Nitric oxide acts as an inducer of Strategy-I responses to increase Fe availability and mobilization in Fe-starved broccoli (Brassica oleracea var. oleracea). Plant Physiol Biochem. 2023;194:182-92.
- [5]Brear EM, Day DA, et al. Iron: an essential micronutrient for the legume-rhizobium symbiosis. Front Plant Sci. 2013;4:359.
- [6]Aznar A, Chen NWG, et al. Immunity to plant pathogens and iron homeostasis. Plant Sci. 2015;240:90-7.
- [7]Rahman MA, Bagchi R, et al. Physiological and molecular characterization of strategy-I responses and expression of Fe-transporters in Fe-deficient soybean. S Afr J Bot. 2022;147:942-50.
- [8]Connolly EL, Campbell NH, et al. Overexpression of the FRO2 ferric chelate reductase confers tolerance to growth on low iron and uncovers posttranscriptional control. Plant Physiol. 2003;133:1102-10.
- [9]Lee K-W, Rahman MA, et al. Nitric oxide-induced proteomic analysis in rice leaves. Plant Biotechnol Rep. 2019;13:375-87.
- [10]Zhai L, Xiao D, et al. Nitric oxide signaling is involved in the response to iron deficiency in the woody plant Malus xiaojinensis. Plant Physiol Biochem. 2016;109:515-24.
- [11]Rahman MA, Kabir AH, et al. Nitric oxide prevents Fe deficiency-induced photosynthetic disturbance and oxidative stress in alfalfa by regulating Fe acquisition and antioxidant defense. Antioxidants 2021;10:1556.
- [12]Hansen NC, Jolley VD, et al. Iron deficiency of soybean in the North Central U.S. and associated soil properties. Soil Sci Plant Nutr. 2004;50:983-7.
- [13]Hoagland DR, Arnon DI. The water-culture method for growing plants without soil. Circular Cali Agric Exp Stat. 1950;347:32.
- [14]Rahman MA, Song Y, et al. Mechanistic basis of silicon mediated cold stress tolerance in alfalfa (Medicago sativa L.). Silicon. 2023.
- [15]Livak KJ, Schmittgen TD. Analysis of relative gene expression data using real-time quantitative PCR and the 2−ΔΔCT method. Methods. 2001;25:402-8.
- [16]Petrou K, Trimborn S, et al. The impact of iron limitation on the physiology of the Antarctic diatom Chaetoceros simplex. Mar Biol. 2014;161:925-37.
- [17]Eichert T, Peguero-Pina JJ, et al. Effects of iron chlorosis and iron resupply on leaf xylem architecture, water relations, gas exchange and stomatal performance of field-grown peach (Prunus persica). Physiol Plant. 2010;138:48-59.
- [18]Astolfi S, Celletti S, et al. Interaction Between Sulfur and Iron in Plants. Frontiers in plant science. 2021;12:670308.